Over a century ago, Albert Einstein suggested a revolutionary theory of gravity that still astounds researchers with its accuracy.
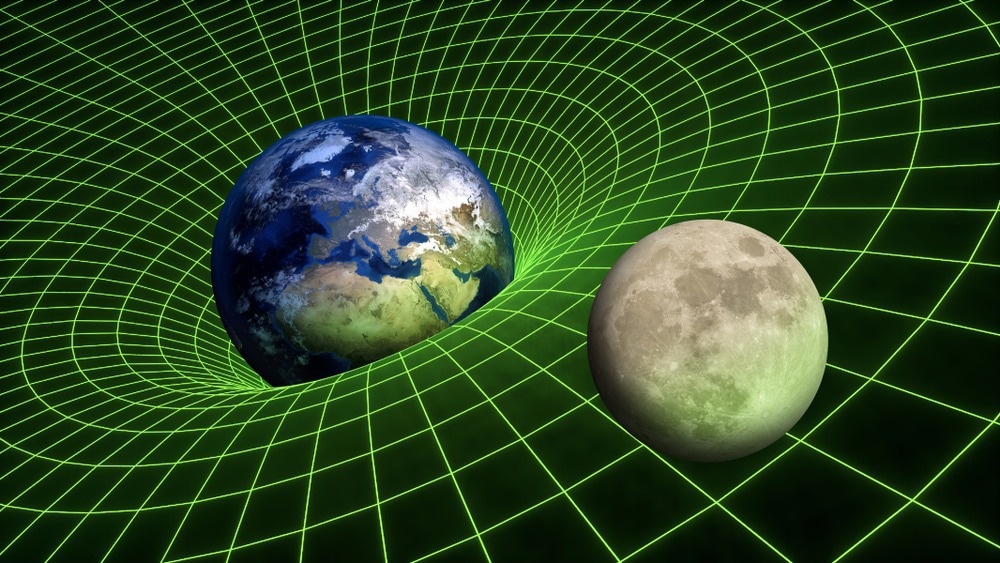
Image Credit: canbedone/Shutterstock.com
Up until 1915, Newton’s theory of gravity had for hundreds of years been the most robust theory of gravity known to man. In fact, the theory is still sufficient for most purposes such as calculating the rate of acceleration a falling object will experience or even for sending rockets into space or to the moon.
However, there remained things that astronomers and other scientists observed that Newton’s theory could not explain. For instance, why did the gravitational mass of a body just happen to be the same as its inertial mass, meaning that objects in freefall all accelerate towards the ground at the same rate? Or why did the motion of Mercury diverge from Newton’s laws of gravity? The planet demonstrates precession effects that prevent its orbit from closing a loop.
Perhaps most significantly, though Newton knew gravity was a force acting between two bodies, his theory did not posit a mechanism through which this force was acting.
In 1915, Einstein would build upon Newton’s theory with his own theory of gravity — general relativity — that would not just address these lingering issues but would grant physicists a renewed picture of the Universe.
Gravity would go from a force acting between two bodies to draw them together to an effect that arises from the influence of mass on the very fabric of space and time.
General relativity would also introduce concepts that have become foundational in science, unthought of in Newton’s theory. The first theoretical seeds of black holes and gravitational waves were planted by the mathematics of general relativity.
That means that the discovery of these elements of the Universe could themselves be considered evidence of the theory’s accuracy.
Yet, researchers have gone further. Tests to confirm Einstein’s most revolutionary theory have moved beyond the limits of Earth to encompass observations of distant events and objects.
It is only fitting that the most robust theory we have of the cosmically massive should find objects like neutron stars, black holes, and even entire galaxies to be the ideal laboratories.
Even with total faith in Einstein, it is still extraordinary just how right he got it when he hit upon general relativity. Even 100 years later, no theory of gravity has surpassed it, and experiments to test its predictions still confirm them to a stunningly high degree of accuracy. While other theories of physics have changed or have been supplanted, increasingly robust observations of the Universe haven’t forced general relativity to change one iota.
The key to understanding how general relativity can be tested and why this has to be done on a cosmic scale lies in understanding what the theory predicts.
What is the Theory of General Relativity and What Did it Predict?
One of the alternative names for the theory of general relativity really sums up its predictions in a nutshell: the geometric theory of gravity.
This is because general relativity predicts that objects of mass cause a curvature of the fabric of space and time, which Einstein united into a single entity called spacetime in 1905 as part of his theory of special relativity. The greater the mass, the more it curves spacetime.
A common analogy used to describe this is a stretched rubber sheet with balls of increasing mass placed upon it. The greater the mass of the ball, the larger the dent in the rubber sheet it causes. A tennis ball causes a slight dip, and a bowling ball an extreme dip.
Now consider what would happen if we roll a marble past these objects. The path of the marble would be diverted by the curvature. The bigger the ‘dent’, the more extreme the diversion.
That is analogous to the effect of gravity. Earth orbits the sun, as do all the other planets in the solar system, because of the localized curvature our star causes on spacetime.
It isn’t just planets that follow these curved paths — or geodesics — through spacetime, however. Light is also diverted by the influence of tremendous mass on spacetime.
Theoretical physicist Frank Wheeler describes the implications of general relativity in 12 words: “Spacetime tells matter how to move; matter tells spacetime how to curve.”
However, the phenomena that emerge from this simple statement when applied to the Universe are anything but simple, and they are the key to proving general relativity correct.
Proving Einstein Right With Gravitational Lensing
Just like the curvature of space diverting the straight path of a planet into an orbit around a star, light’s path is also diverted by objects of tremendous mass like galaxies. This gives rise to a powerful phenomenon in astronomy called gravitational lensing.
To see the results of gravitational lensing consider a distant light source like a galaxy. In between this source galaxy and Earth is another galaxy — which acts as a gravitational lens. The light from the source galaxy is curved as it passes the lens galaxy which has the effect of shifting the source’s position in the sky to an apparent position.
The phenomenon of gravitational lensing doesn’t just amplify the light from distant sources; because light from the source can take different paths around a lens object, this can make it arrive at different times. This creates the illusion of a single object like a star or distant galaxy appearing in the night sky multiple times.
These illusions can take a variety of shapes such as Einstein rings or Einstein crosses.
Gravitational lensing provided the first categorical evidence that general relativity was the right recipe for gravity in 1919. Einstein had already demonstrated the orbit of Mercury could be explained by general relativity, but the scientific community at large would need more.
English astronomer Arthur Eddington, an early champion of the theory, realized that the curvature of light predicted by general relativity could be demonstrated during a solar eclipse.
While the sun blotted out the stars behind it —the bright Hyades star cluster — they should be visible and their positions in the sky should be shifted to an apparent position. Eddington traveled with a team to Príncipe, an island off the African coast, while Andrew Crommelin of the Royal Greenwich Observatory led a second expedition to Sobral in Brazil.
Comparing images taken by both teams during the eclipse, Eddington confirmed a change in position of 1.75 arc seconds — almost exactly the shift in position that general relativity predicted.
Further testing of general relativity would stall after this, however, until the 1960s when telescope technology improved enough to allow detailed observations outside of the solar system.
This modern testing would often involve an even more shocking and violent phenomenon predicted by general relativity.
Black Holes Are Such a Drag
General relativity has already explained the effect that an object of great mass has on spacetime, but this is a limited picture, as most objects don’t sit in spacetime passively.
Indeed, general relativity accounted for what would happen to truly massive objects when they are spinning. This gives rise to an effect called frame-dragging — or Lense-Thirring precession.
This is when a massive object like a rotating black hole — formally known as a Kerr black hole — twists the very fabric of spacetime and drags it along with it. To imagine this, picture a bowl of honey with food coloring dropped in it. Placing a spoon at the center and turning churns the honey and twists the food coloring around the central region.
This ‘churning’ of spacetime that is created means that there should be an area at the edge of any Kerr black hole in which any object or particle would find it impossible to sit still.
The phenomenon should be particularly powerful around neutron stars, which, like black holes, form when massive stars collapse, but are also known to spin incredibly rapidly.
In early 2020, a team of researchers led by scientists from the Max Planck Institute, Bonn, Germany, observed the swirling of spacetime around a neutron star and white dwarf binary pair located
The white dwarf in this system — named PSR J1141–6545 — has been sped up so much by the transfer of mass from its neutron star partner that its rotational period is just one minute. This means that the frame-dragging effect which would not normally be visible around a white dwarf could be seen in this system by the team.
Our instruments have now become so precise that we are even capable of detecting frame dragging around Earth. While the Gravity Probe B instrument uses precision gyroscopes to measure this effect around our planet, laser-ranging satellites LAGEOS 1 & 2 and LARES measure the resultant slow precession of the orbital plane of the satellites in the direction of Earth’s rotation.
These instruments have confirmed frame-dragging within 2 percent of that which was predicted 100 years ago by Einstein.
Einstein Proved Right… Again and Again
Perhaps the most shocking thing about general relativity that scientists have uncovered in the century since Einstein first put it forward is just how accurate it is. The theory has passed every test with flying colors.
This was never more evident than in May of 2022 when astronomers from the Event Horizon Telescope (EHT) project revealed the first direct image of the supermassive black hole at the heart of the Milky Way — Sagittarius A*(Sgr A*).
The glowing ring that encloses the black hole and the physics around it match to a high degree predictions made by Einstein in 1915 before he even knew black holes exist or that one lies at the center of our own galaxy.
Institute of Astronomy and Astrophysics and EHT project researcher Geoffrey Bower from the Institute of Astronomy and Astrophysics remarked at the time: “We were stunned by how well the size of the ring agreed with predictions from Einstein’s theory of general relativity.”
Sgr A* has already been used to test the predictions of general relativity via observations made of the star — S2 — which orbits close to the supermassive black hole.
This star’s orbit traces out a rosette-like shape as it orbits Sgr A* at a blisteringly fast speed of 30 million feet per second. This is a result of precession effects similar to those of Mercury, which Newton’s theory couldn’t explain way back in 1915. It also perfectly conforms to the predictions of general relativity.
Physicists will continue to push the limits of general relativity, attempting to find the limit at which Einstein’s magnum opus fails to accurately describe the Universe.
That is not to say it is perfect, however. Physicists are yet to find a way to unite this theory of the massive with science’s best theory of the subatomic — quantum physics. Perhaps when we finally hit upon a ‘quantum theory of gravity’ Einstein’s general relativity, like Newton’s theory of gravity, may be surpassed.
Until that day, it is only right to marvel at the accuracy of this theory that has passed every conceivable test.
More from AZoQuantum: James Webb Space Telescope Spots Light From The Oldest Galaxy Ever Found
References and Further Reading
Lambourne. R., [2010], Relativity, Gravitation, and Cosmology, Cambridge University Press
Cheng, T-P., [2005], Relativity, Gravitation, and Cosmology, Oxford University Press Gravitational Waves
Maggiore. M., [2018], Gravitational Waves: Volume 2 Astrophysics and Cosmology, Oxford University Press
Disclaimer: The views expressed here are those of the author expressed in their private capacity and do not necessarily represent the views of AZoM.com Limited T/A AZoNetwork the owner and operator of this website. This disclaimer forms part of the Terms and conditions of use of this website.