Quantum sensing technologies (QST) use quantum effects, endowing the sensors with exceptional abilities.1 In recent years, quantum sensing has emerged as a potentially influential and integral part of Quantum Information Systems, offering major benefits across various economic sectors.2
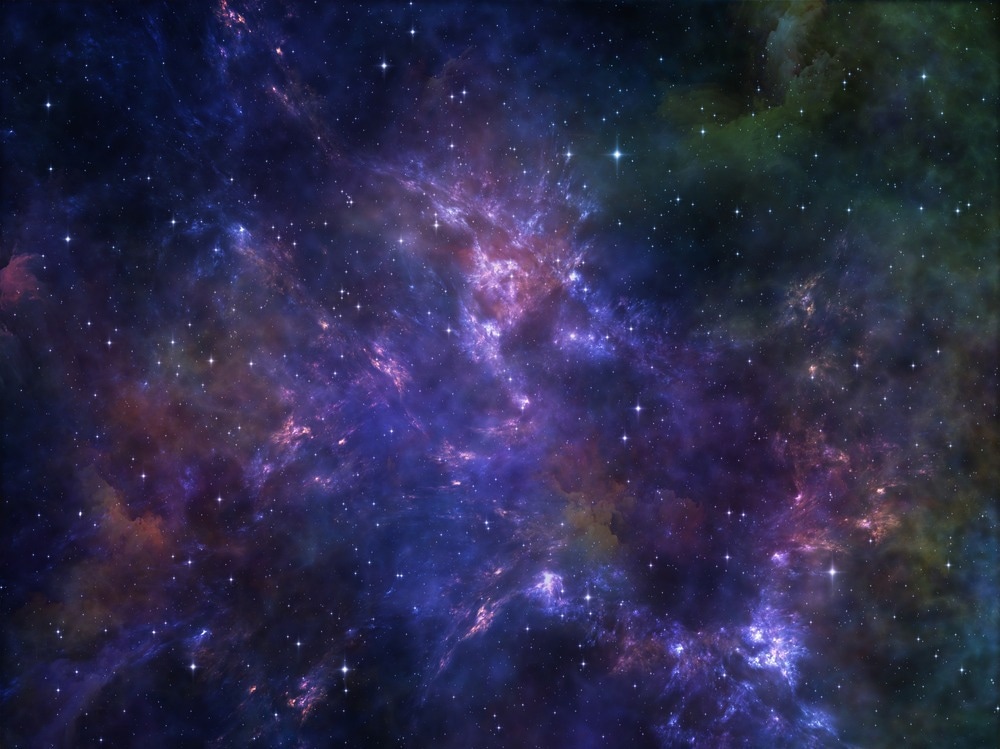
Image Credit: agsandrew/Shutterstock.com
As quantum systems are highly sensitive to external changes, quantum sensors can monitor various physical characteristics with greater precision, sensitivity, and accuracy. These systems leverage quantum mechanics concepts, such as superposition and quantum entanglement, for superior sensing mechanisms.
The benefits of quantum sensors have accelerated their utilization in space exploration, particularly for navigational purposes, vehicle positioning, gravitational mapping, and climate studies.
As quantum sensing technology continues to progress, it is poised to play a central role in addressing the majority of the challenges the space industry faces now and in the future.
The Principles of Quantum Sensing
Superposition and quantum entanglement are foundational principles that elevate quantum sensing systems as the preferred choice for modern applications.3 Quantum sensing utilizes the sensitivity of quantum states to detect small environmental changes, thereby gathering more detailed information about the natural world.
In this field, scientists are using quantum entanglement between nuclear and electronic states or between electronic states of unique materials.4
Quantum sensors require methods for establishing the initial sensor state and maintaining coherent quantum states for durations long enough to enable precise sensing and read-out. Meeting these criteria, especially the creation of a well-defined, two-level quantum system, facilitates the use of quantum entanglement to enhance sensor performance in multi-sensor measurements.
Quantum entanglement is a phenomenon where the quantum states of systems are inherently interconnected and unable to be described independently. Arrays of entangled sensors have the potential to exceed the standard quantum limit.
The superposition principle in quantum mechanics gives rise to non-classical features like quantum coherence and entanglement.5
The two-mode squeezed state (TMSS) is an entangled state involving two bosonic modes. This correlation displays Bell nonlocality, crucial for implementing quantum teleportation, detecting weak fields like gravitational waves, and significantly contributing to quantum sensing.6
Another interesting non-classical state is the cat state, a quantum superposition of two opposite coherent states. These cat states have been widely used in quantum sensing.
Over the past decade, quantum sensing has gained popularity for infrared spectral range measurements. Information from the sample in the desired spectral region is transferred through bi-photon correlations to another spectral range, where highly sensitive detectors are accessible.7
This approach is particularly advantageous for terahertz radiation, as semiconductor detectors are unavailable for this range. As a result, quantum sensing has recently been applied for terahertz radiation detection in various industries. This involves bombarding the sample with terahertz protons, allowing quantum sensors to obtain information about the sample's thickness and geometry non-destructively.8
Quantum sensing is emerging as a promising method for space-borne Earth Observation (EO), potentially offering greater accuracy, sensitivity, and stability than traditional EO instruments.
Several quantum sensors have been developed and tested in ground-based experiments; some are even commercially available. Other technologies, like cold-atom interferometers, magnetometers, Rydberg receivers, and quantum lidar, could enhance EO capabilities. These technologies have been extensively utilized for EO missions at the European Space Agency.9
Detecting Gravitational Waves Using Quantum Sensing Technology
Combining Einstein's theory of gravity with quantum mechanics remains one of modern physics’ central unresolved issues. The absence of experimental proof for quantum gravity is a significant hurdle.
It is hypothesized that the quantization of gravity leads to gravitons, particles with discrete energy that create gravitational waves. However, until recently, the detection of gravitons has been unattainable.
In a new study, researchers have demonstrated that signs of individual gravitons can be detected in laboratory experiments through a gravito-phononic version of the photoelectric effect and continuous quantum measurement of energy states.10
In general relativity, gravity interacts via the non-linear Einstein equations, linking space-time geometry to matter. The team has identified two enhancement mechanisms for detecting single graviton signatures.
The first focuses on stimulated processes in a gravitational wave backdrop, potentially leading to single graviton transitions. The second involves considering massive quantum systems set up in quantum states at macroscopic mass scales, coupled with ongoing measurements of individual energy quanta.
Despite interacting with essentially classical waves containing more than 1036 gravitons, the interaction remains weak enough that only single quanta are exchanged within relevant time frames. Achieving this requires parameters that are challenging but feasible.
By correlating measurements with classical LIGO detection events, it becomes feasible to provide the initial evidence of gravitons. While this approach could also function at higher frequencies, the uncertainty regarding potential sources persists.
Analogous to the electromagnetic photoelectric effect, such detections would represent the first evidence of gravitons, marking the most convincing experimental indication of quantum gravity to date.
Can Quantum Sensing Be Used for Dark Matter Detection?
The nature of dark matter (DM) is among the most complex puzzles in modern physics. Experts have identified potential candidates for dark matter detection, including weakly interacting massive particles (WIMPs), with mass values ranging from a few GeV to 100 TeV.
Next-generation dark matter detectors are being designed to search for WIMPs, aiming to detect coherent scattering from solar neutrinos.11 This requires an efficient tool for discriminating between background signals and actual detections.
Directional detectors are instrumental in enhancing sensitivity to WIMP DM despite the unavoidable neutrino background.
Wide-bandgap semiconductors emerge as a promising option for directional detection within high-density target materials. These detectors operate in a hybrid mode, detecting WIMP or neutrino-induced nuclear recoils in real time through the collection of charge, phonon, or photon signals.
However, the directional signal is etched as a lasting sub-micron damage track within the lattice structure. This signal can be analyzed using various atomic physics techniques, from point defect quantum sensing to X-Ray microscopy.
Researchers have envisioned a solid-state detector for directional WIMP detection operating in a hybrid mode.12 This proposed detector method combines real-time event registration, 3D directional information, and high-density target material.
In a solid-state crystalline detector, a WIMP recoil would transfer significant kinetic energy to a target nucleus, displacing it from its lattice site. This displaced nucleus would initiate a series of secondary recoils, resulting in a distinctive damage track composed of lattice vacancies, interstitial nuclei, and altered bonds.
Hybrid detectors of this nature could identify potential WIMP events using established methods for semiconductor solid-state detectors, including charge, phonon, and scintillation detection.
Quantum Sensing Technology: Challenges and Innovations
Despite the resources available for quantum sensing and the scientific community's interest, several barriers hinder the development of quantum sensing technology for space applications.
One challenge is the lack of familiarity with the term "quantum," leading to misconceptions about its capabilities. The association of "quantum" with novelty and complexity may overshadow the nuanced strengths, weaknesses, and maturity levels of specific quantum sensing systems.
The concentration of experienced quantum science and technology professionals in laboratories and research facilities, rather than in leadership roles within agencies such as NASA, also presents communication challenges between scientists and supervisors.13
While understanding traditional technologies like telescopes or space shuttles is straightforward, grasping quantum sensing technology is less intuitive. Consequently, supervisors may hesitate to allocate additional resources or grant researchers the autonomy to explore quantum sensing approaches.
While quantum sensing has the potential for a wide range of applications, determining its feasibility requires extensive research and development. The investment required to determine the most effective application of quantum technology may conflict with the mission-driven structure of projects, which typically operate under strict timelines.
However, the future of quantum sensing does look bright. SBQuantum, a Canadian company, has created a magnetometer that utilizes the superposition of quantum states to improve its sensitivity to magnetic fields.14 This innovation marks the company as one of the first to develop quantum magnetometers made from diamond among various startups and organizations.
These quantum sensing devices can provide vector measurements of both the strength and direction of Earth's magnetic field. SBQuantum's diamond magnetometer harnesses quantum properties to minimize drifts, such as those caused by temperature limitations, which can distort readings obtained from current classical technologies.
The application of quantum technology to improve sensor efficiency is poised to play a crucial role in advancing space exploration.
More from AZoQuantum: Advancing Technology with 2D Quantum Materials
References and Further Reading
[1] Granelli, F., et al. (2022). A novel architecture for future classical-quantum communication networks. Wireless Communications and Mobile Computing. doi.org/10.1155/2022/3770994
[2] Kantsepolsky, B., et al. (2023). Exploring quantum sensing potential for systems applications. IEEE Access. doi.org/10.1109/ACCESS.2023.3262506
[3] IZAK Scientific. (2024). Quantum Sensing: Unveiling the Future of Precision Measurement. [Online] IZAK Scientific. Available at: https://izakscientific.com/quantum-sensing-unveiling-the-future-of-precision-measurement/ (Accessed on 31 March, 2024).
[4] Yu, C., et al. (2021). A molecular approach to quantum sensing. ACS central science. doi.org/10.1021/acscentsci.0c00737
[5] Streltsov, A., et al. (2017). Colloquium: Quantum coherence as a resource. Reviews of Modern Physics. doi.org/10.1103/RevModPhys.89.041003
[6] Cardoso, F., et al. (2021). Superposition of two-mode squeezed states for quantum information processing and quantum sensing. Physical Review A. doi.org/10.1103/PhysRevA.103.062405
[7] Chekhova, M., et al. (2016). Nonlinear interferometers in quantum optics. Advances in Optics and Photonics. doi.org/10.1364/AOP.8.000104
[8] Kutas, M., et al. (2020). Terahertz quantum sensing. Science Advances. doi.org/10.1126/sciadv.aaz8065
[9] Strangfeld, A., et al. (2023). Quantum sensing for Earth observation at the European Space Agency: latest developments, challenges, and future prospects. Sensors, Systems, and Next-Generation Satellites XXVII. doi.org/10.1117/12.2678430
[10] Tobar, G., et al. (2023). Detecting single gravitons with quantum sensing. arXiv preprint arXiv:2308. doi.org/10.48550/arXiv.2308.15440
[11] Particle Data Group., Zyla, P., Barnett, R., et al. (2020). Review of particle physics. Progress of theoretical and experimental physics. doi.org/10.1093/ptep/ptaa104
[12] Ebadi, R., et al. (2022). Directional detection of dark matter using solid-state quantum sensing. AVS Quantum Science. doi.org/10.1116/5.0117301
[13] Frieling, H. (2022). A Strategic Lens On Quantum Sensing For Space Applications. [Online] NASA: Office of Technology, Policy and Strategy. Available at: https://ntrs.nasa.gov/api/citations/20220009225/downloads/Frieling_STRIVESReport.pdf (Accessed on 1 April, 2024).
[14] SBQuantum. (2023). SBQuantum to test quantum magnetometer in space - designed to map Earth’s magnetic field. [Online] EurekAlert . Available at: https://www.eurekalert.org/news-releases/1001911 [Accessed on 1 April, 2024].
Disclaimer: The views expressed here are those of the author expressed in their private capacity and do not necessarily represent the views of AZoM.com Limited T/A AZoNetwork the owner and operator of this website. This disclaimer forms part of the Terms and conditions of use of this website.