Thus far, time crystals and discrete time crystals, exotic phases of matter, have only been understandable with quantum physics. New research suggests that a simpler “classic” approach might unlock the secrets of these systems.
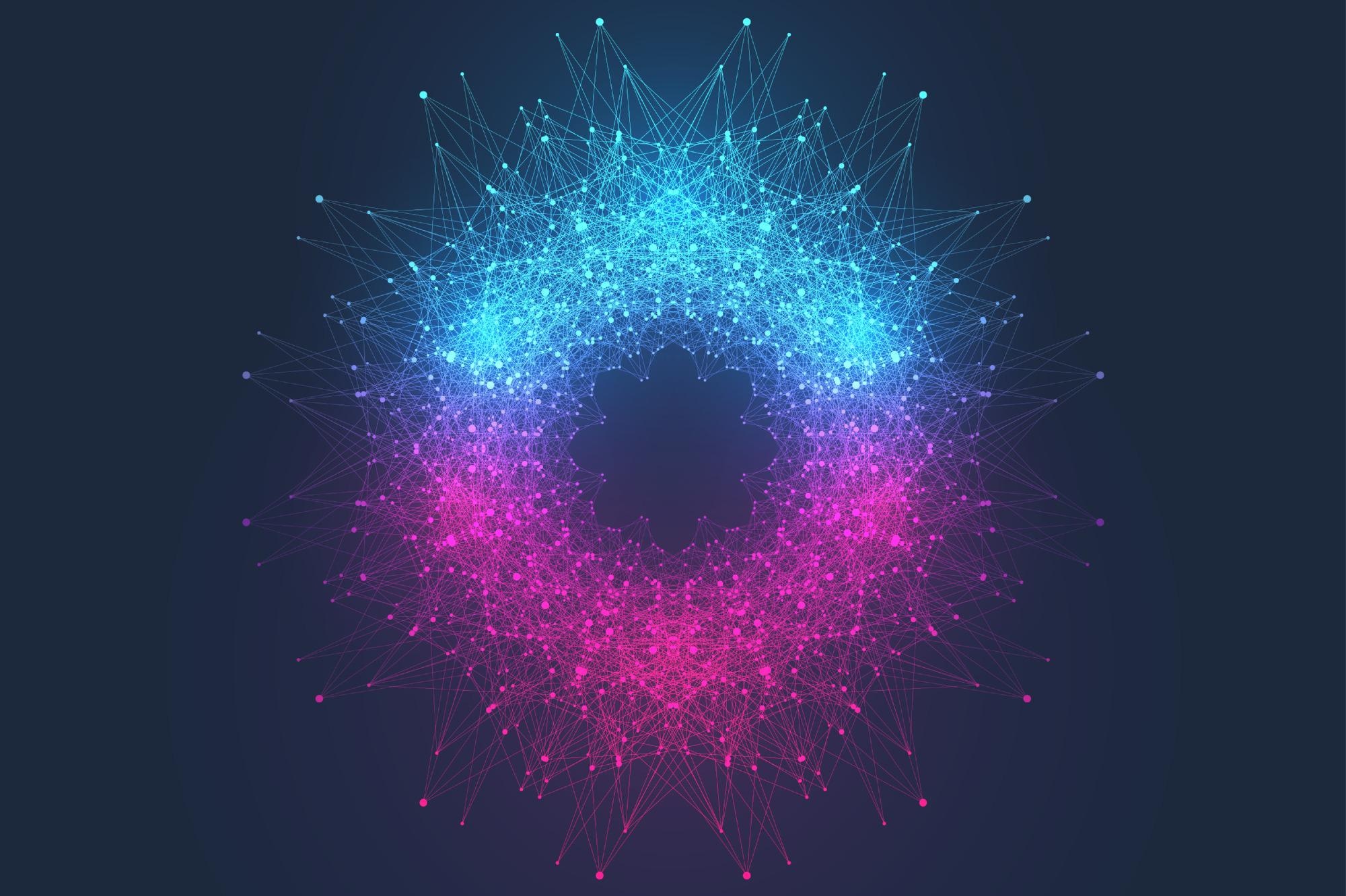
Image Credit: BAIVECTOR/Shutterstock.com
Time crystals are a state of matter in which the particles are in their lowest energy state and yet still remain in a state of repetitive motion. This means that this system cannot lose energy as it already exists in what is known as a ground state.
This makes time crystals a new and unique form of matter, possessing motion without energy. Time crystals, not actual crystals at all but particles in a lattice-like arrangement, were first hypothetically suggested by American theoretical physicist Frank Wilczek in 2012, with atoms arranged not just in space, but in space and time.
The creation of a time crystal would have significant implications for physics as it represents matter that spontaneously breaks so-called time-translation symmetry (TTS) — the concept that the laws of physics are unchanged — invariant — under such a transformation.
Such a creation could even challenge the second law of thermodynamics, the idea that unusable energy builds within a closed physical system. Understanding this new phase of matter could also represent a step towards the control of complex many-body systems, a long-standing goal with various potential applications, such as simulations of complex quantum networks.
While time crystals have been hypothetical for a long time, an increasing number of researchers are beginning to claim to have generated an actual time crystal. Some meet more criteria of an actual time crystal than others.
A recent paper published on the repository ArVix¹ details the production of a state of matter matching many criteria of a time crystal, with Google’s Sycamore quantum computer. This research followed a study by another team of researchers that they had created a time crystal sealed with a diamond².
The development demonstrates the capacity of a quantum computer to model quantum systems accurately. The difficulty is that currently, the only way to model a time crystal is by taking a quantum approach.
As quantum systems comprised of a large number of particles can only be modeled accurately by a quantum computer, that severely limits physicists’ ability to model time crystals.
Further Reading: How Small Can We Make Quantum Computers?
But, this could be about to change. Researchers from the University of Cambridge in the United Kingdom believe that they have a way to study time crystals with classical physics. This means, as a consequence that this novel state of matter could potentially be modeled with a classical computer.
A Classic Approach to a Quantum Problem
In two papers published in the journals Physical Review Letters and Physical Review B the researchers describe the use of computer modeling to study potential new phases of matter known as prethermal discrete time crystals (DTCs).
The researchers, including the first author of both papers, a Ph.D. candidate in Cambridge’s Cavendish Laboratory Andrea Pizzi, had initially believed that the only way to study DTCs was with the discipline of quantum physics.
They were surprised, therefore, to discover that DTCs could also be modeled with the far simpler framework of classical physics.
DTCs are complex physical systems, remarkable because just as how “space crystals” break space-translational symmetry simply because their structure isn’t the same everywhere in space, DTCs break a distinct time-translational symmetry. This is because, when they are ‘shaken’ periodically, their structure changes with every ‘push’.
Pizzi compares this to pushing a child on a swing. When one child is pushed the system is simple eventually returning to an equilibrium position. But imagine a row of connected swings with children holding hands.
A simple push now creates a more complex and difficult to predict system. The multiple swing system is far more interesting also as different and less obvious behaviors emerge from a simple push. A DTC is analogous to the multiple swing system, with interacting spins — a type of quantum mechanical magnetic angular momentum — reacting to a single push creating complex and difficult to predict behaviors.
One of the behaviors of DTCs that demonstrate this unusual and unpredictable behavior as they don’t heat as quickly as could be expected. Instead, they exhibit time-crystalline behavior for extended periods. Additionally, the faster DTCs are shaken, the longer they survive.
The research team headed up by Pizzi discovered that they could model DTCs without recourse to complicated quantum physics and relying on the massive computing power needed to do this.
Instead, they found a classical solution to DTCs studying interacting spins under the action of a periodic magnetic field, like a parent providing a push on a swing, using classical dynamics.
This approach demonstrated the properties of prethermal DTCs showing that for a prolonged period of time the magnetization of the system oscillates with a period larger than that of the drive.
The method developed by the team should allow researchers to look at larger and more complex systems than DTCs, showing clearly what is happening in those systems. And excitingly, this could be done with a classical, non-quantum approach.
Sources
Google Quantum AI and collaborators, [2021], “Observation of Time-Crystalline Eigenstate Order on a Quantum Processor.” [https://arxiv.org/pdf/2107.13571.pdf]
Randall. J., Bradley. C. E., van der Gronden. F. V., et al, [2021], “Observation of a many-body-localized discrete time crystal with a programmable spin-based quantum simulator.” [https://arxiv.org/pdf/2107.00736.pdf]
Pizzi. A., Nunnenkamp. A., Knolle. J., et al., [2021], ‘Classical Prethermal Phases of Matter,’ Physical Review Letters, [https://journals.aps.org/prl/abstract/10.1103/PhysRevLett.127.140602]
Pizzi. A., Nunnenkamp. A., Knolle. J., et al., [2021], ‘Classical approaches to prethermal discrete time crystals in one, two, and three dimensions,’ Physical Review B, [https://journals.aps.org/prb/abstract/10.1103/PhysRevB.104.094308]
Disclaimer: The views expressed here are those of the author expressed in their private capacity and do not necessarily represent the views of AZoM.com Limited T/A AZoNetwork the owner and operator of this website. This disclaimer forms part of the Terms and conditions of use of this website.