A new frontier of experimental physics is emerging. Just as the first X-ray telescope revolutionized observational astronomy by allowing scientists to probe physics that did not lie within the visible portion of the electromagnetic spectrum, gravitational wave detectors allow the study of the Universe in a separate, previously unimagined spectrum – the gravitational wave (GW) spectrum.
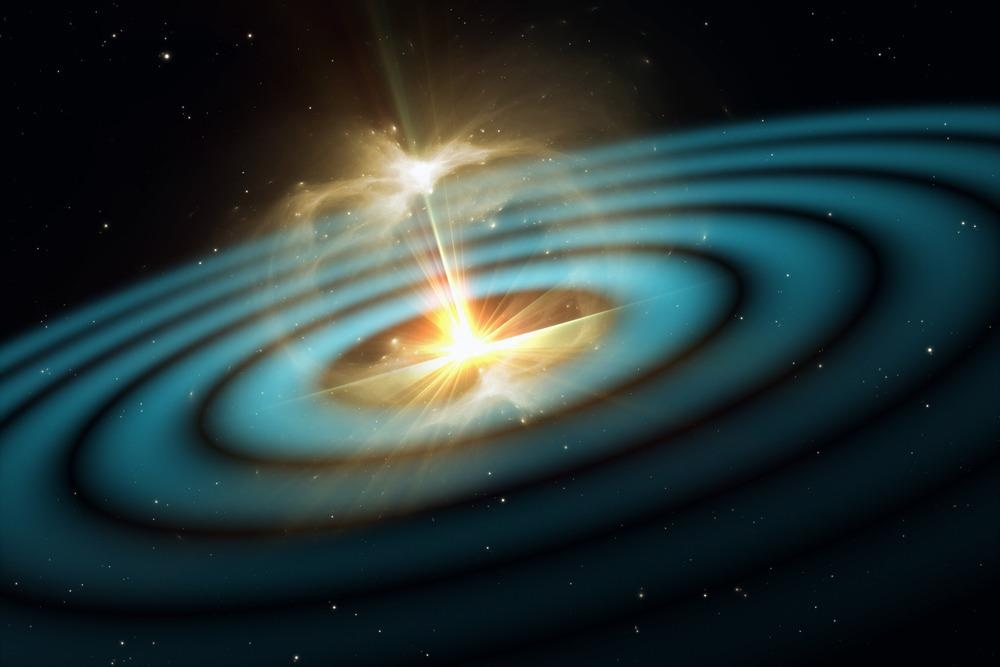
Image Credit: Jurik Peter/Shutterstock.com
The motion of all forms of mass/energy generate GWs, which propagate unimpeded through space and time. GW’s are measured as a frequency (Hz). Proposed originally in Einstein’s General Theory of Relativity (GR), GWs can be studied to provide further evidence for GR, or, if necessary, to modify GR to suit observations. Thus far, GR has passed all tests in the weak-field (i.e., at large distances from massive objects).
A highly sensitive instrument, known as an interferometer, is used to measure the passing of GWs. An interferometer is a specially designed network of mirrors and perpendicular lasers positioned at precise distances away from each other.
As a GW passes through an interferometer, space is distorted in such a way that the distance between the extremities of the interferometer oscillates on a nanoscopic scale. For reference, the most advanced interferometer on Earth can detect a change in distance between its mirrors of 1/10,000th the width of a proton.
The concept of superposition is applied to lasers to gauge these extremely small changes in distance. In theory and in practise, the intensity of the laser oscillates as the distance between the extremities oscillates. A photodetector is used to measure the changes in intensity of the laser system.
2016 saw the verification of the first observed gravitational wave, GW150914, detected 5 months earlier by the Laser Interferometer Gravitational-Wave Observatory (LIGO), based at the Hanford and Livingston sites, separated by 3000km.
The LIGO Group detected the merging of two stellar-mass black holes, followed by the subsequent creation of a resulting single black hole. The unmistakeable results published by The LIGO Group sent shock waves through the astrophysics community; this was the beginning of a new chapter in observational astronomy.
The progression of science is inevitable. A founding principle of interferometry is that larger interferometers with stronger lasers will measure lower frequencies.
The two interferometers used by LIGO, currently the most advanced terrestrial-based interferometers, each have two 4km long perpendicular arms and use lasers that are 200W. This allows for measurements within the 32Hz to 4kHz band of the GW spectrum.
However, the problem is that terrestrial-based interferometers can only be so large, as they must be kept away from man-made disturbances to pick up as little noise as possible.
An ambitious project, led by the ESA and backed by NASA, is in the process of manifesting. The Large Interferometer Space Antenna (LISA) mission, set to launch in the early 2030’s [5], will be the first ever space-based GW detector.
A gigametre-scale space-based GW detector would allow for the probing of the GW spectrum between 0.1mHz and 1Hz. This previously inaccessible frequency band is expected to host an extensive list of astrophysical systems that may not be visible using terrestrial-based interferometers or instruments that measure in the EM spectrum.
The 8 science objectives of the LISA mission are outlined in the LISA Science Requirements Document.
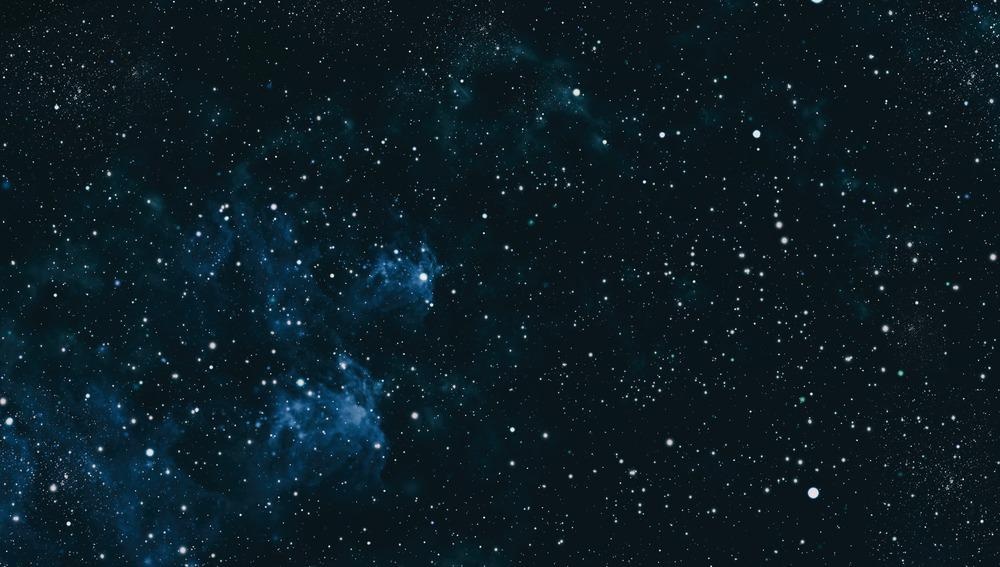
Image Credit: Zakharchuk/Shutterstock.com
The first four objectives are concerned with observing astrophysical systems, including studying the formation and evolution of compact binary stars in the Milky Way; the origin and growth history of massive black holes and probing the dynamics within extreme mass-ration inspirals.
A multitude of cosmological sources have also been proposed to be detected by a space-based GW detector. Warped miniature dimensions, cosmic strings, self-interacting dark matter and boson stars are amongst a long list of potential GW source candidates that a space-based GW detector could observe.
Furthermore, LISA allows scientists to test GR in the strong-field regime (i.e., close to massive objects) and, more generally, test the accuracy of our current large-scale Universe predictions by directly studying the early Universe.
In predictions that go beyond the standard model, the physical vacuum of the very early Universe may have undergone one or more first-order electroweak phase transitions [9], generating enormous GWs that have been traveling throughout the Universe ever since.
These monstrous GWs, over the course of billions of years, have been calculated to now lie within the mHz portion of the GW spectrum [10]. This calculation comes from the idea that GWs become redshifted with the expansion of the Universe just as EM waves become redshifted.
At the mHz frequency band, a space-based interferometer will also encounter stochastic GW backgrounds. One would be quick but wrong to assume this is useless noise that should be omitted from results. Key information regarding the physics of the very early Universe may be encoded within these GWs.
To conclude, almost all of the Universe is electromagnetically dark. However, the Universe does have an unexpectedly loud GW sky at mHz frequencies.
A gigametre-scale space-based interferometer that is able to observe within the mHz frequency band, such as the ESA’s LISA, would allow for immense discoveries concerning astrophysical systems. With an ambitious (yet achievable) launch date of within the early 2030’s, the coming decade should see many advancements in the fields of astronomy and laser technology.
References and Further Reading:
The eLISA Consortium. (2013). The Gravitational Universe, arXiv:1305.5720. https://arxiv.org/abs/1305.5720
LIGO Lab | Caltech. (2021). Facts. [online] Available at: https://www.ligo.caltech.edu/page/facts
The LIGO Scientific Collaboration. (2019). The Virgo Collaboration, All-sky search for short gravitational-wave bursts in the second Advanced LIGO and Advanced Virgo run, arXiv:1905.03457 [gr-qc], Phys. Rev. D100, 024017 https://arxiv.org/abs/1905.03457
G.M. Harry. (2010). LIGO Scientific Collaboration, Advanced LIGO: the next generation of gravitational wave detectors, Class. Quantum Grav. 27 084006, https://iopscience.iop.org/article/10.1088/0264-9381/27/8/084006
Sci.esa.int. (2021). ESA Science & Technology - LISA. [online] Available at: https://sci.esa.int/web/lisa
J. Baker et al., (2019). The Laser Interferometer Space Antenna: Unveiling the Millihertz Gravitational Wave Sky, arXiv:1907.06482, https://arxiv.org/abs/1907.06482
K. Danzmann et al.,(2019). LISA: Laser Interferometer Space Antenna, arXiv:1702.00786 [astro-ph.IM], https://arxiv.org/abs/1702.00786
LISA Science Requirements Document, (2018). ESA-L3-EST-SCI-RS-001, https://www.cosmos.esa.int/documents/678316/1700384/SciRD.pdf
C. J. Hogan, (1986). Gravitational radiation from cosmological phase transitions, Monthly Notices of the Royal Astronomical Society, Volume 218, Issue 4, https://academic.oup.com/mnras/article/218/4/629/1012412
A.R. Pol et al.,(2020). Numerical simulations of gravitational waves from early-universe turbulence, arXiv:1903.08585 [astro-ph.CO], Phys. Rev. D102, 083512, https://arxiv.org/abs/1903.08585
Disclaimer: The views expressed here are those of the author expressed in their private capacity and do not necessarily represent the views of AZoM.com Limited T/A AZoNetwork the owner and operator of this website. This disclaimer forms part of the Terms and conditions of use of this website.