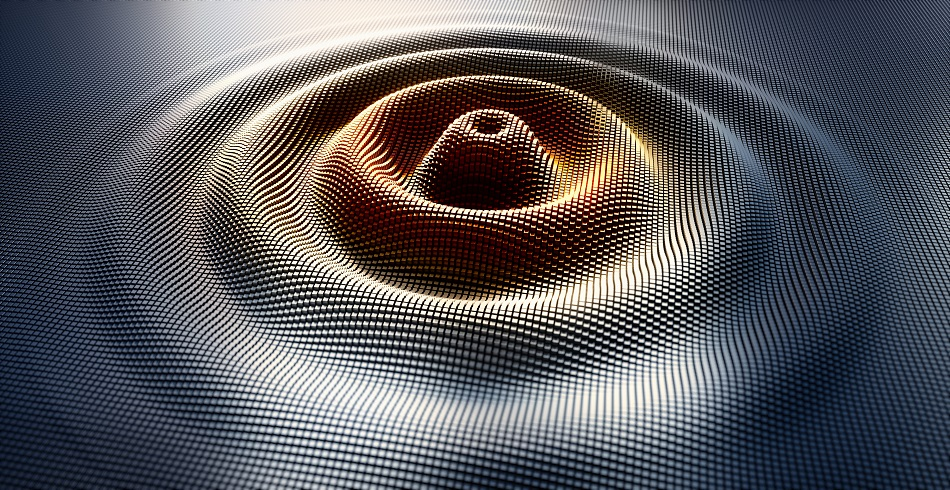
Image Credit: peterschreiber.media/Shutterstock.com
In February 2016, the worldwide scientific community reacted to news that gravitational waves – first predicted by Albert Einstein one hundred years earlier as an inference of his ground-breaking theory of general relativity – had been empirically detected. The detection, made at the paired Laser Interferometer Gravitational-Wave Observatory (LIGO) facilities in Washington and Louisiana in the US, was enabled by advances in our understanding of quantum physics and its subsequent application in the field of interferometry.
Interferometry
The techniques for superimposing (usually electromagnetic) waves and extracting information from the interference this causes is named interferometry.
In most interferometry techniques, a single beam of light is split into two, typically by a partially reflective mirror known as the beam splitter. The two beams are made to follow different optical paths and then combined again at a detector.
The phase difference between the two beams after they have recombined is caused by the different lengths of each optical path. It causes interference, and close analysis of that can provide information about minute differences between the optical paths' lengths.
With some techniques, multiple incoherent light sources are recombined to cause interference in the same way. Some techniques use other forms of electromagnetic waves than light.
The interference is analogous to the cross-hatch pattern of waves on a body of water in gusting wind or with wind and current acting on the water at the same time.
Interference is diagnostic of anything that could alter the optical path of the split light beam (or of other kinds of waves). For example, differences in the refractive index of materials in the optical path can be measured, as well as differences in distance traveled.
Modern sensors can measure interference with extreme precision, and interferometers are used by scientists and the advanced manufacturing industry to calculate the length and shape of optical components with precision to the nanometer. No other instruments exist today that provide the same level of accuracy for length measurements.
In astronomy, interferometry is used in the same way as in manufacturing and materials science, but on a relatively massive scale. Typically, two telescopes are paired so that their signals can be combined. This way, the resolution is equivalent to a telescope whose diameter equals the largest distance between the paired facilities.
Detecting Gravitational Waves
The twin facilities LIGO each have a 4 km long interferometer. They are shaped like the letter L, and they utilize the Michelson–Fabry–Pérot interferometry technique. This combines the Michelson method of sending light on two separate but linear optical paths and back to the detector with the Fabry–Pérot method of bouncing light between thin mirrors in an optical cavity.
In the LIGO facilities, photons are stored for nearly one millisecond in the optical cavity, bouncing between mirrors until they reach the same resonance and can escape. This means that more time is allowed for a disturbance to interact with the light, and enables more sensitivity when low frequencies are used.
Then, they are sent 4 km in perpendicular directions down the arms of the L shaped facilities, and interference is recorded when they return to the crux. The huge distance covered by the split light beams – and the continental gap between the twin facilities – gives LIGO the greatest resolution capacity of any facility of its kind.
On February 11th, 2015, LIGO announced they had detected gravitational waves with their massive interferometer, one hundred years after the phenomenon was first predicted by Albert Einstein. The gravitational wave was caused when two black holes collided 1.3 billion years ago, sending off a faint ripple through the fabric of space-time. The LIGO facilities recorded a distance change one-thousandth of a proton in length, due to the extreme level of resolution enabled by the interferometry techniques employed there.
Disclaimer: The views expressed here are those of the author expressed in their private capacity and do not necessarily represent the views of AZoM.com Limited T/A AZoNetwork the owner and operator of this website. This disclaimer forms part of the Terms and conditions of use of this website.