AZoQuantum had the pleasure of speaking with Dr. Foni Raphaël Lebrun-Gallagher about his team's breakthrough research at the University of Sussex. Their latest research proves the viability of connecting independent ion-trap quantum computer modules via electric fields.
Could you please tell us a little about your background and how you came to be involved with this research?
I grew up between France and Luxembourg and did my undergraduate degree in Physical Sciences at the University of Rennes. I accidentally stumbled across the fascinating world of quantum physics while on an astrophysics research placement at the University of Queensland, Australia, and since then the passion never faded. In 2014 I joined the University of Sussex' Master's program in Quantum Technologies where I found it incredibly exciting that our current level of scientific development not only allowed us to use quantum mechanics to describe the microscopic world around us but also allowed us to engineer useful tools whose inner workings exploit this theory.
While at Sussex, I met Prof. Winfried Hensinger and his cutting-edge research group, who inspired me to join the quest for building practical quantum computers using a levitating array of trapped ions. During my Ph.D. studies under his supervision, and for now more than five years, I have been working on developing solutions to overcome the outstanding physics and engineering obstacles that are in the way of scaling up quantum computers
For these machines to be able to tackle meaningful, wide-ranging problems, quantum computers would need to scale to qubits numbers that are in the millions. Now, this can be quite the disenchanting realization as, by comparison, today’s most advanced quantum computers are only capable of hosting a handful of qubits, with qubit numbers typically below 100.
From the first day, the goal of the research team was very clear. We needed to develop practical techniques and hardware, that would allow quantum computers to no longer be constrained to such numbers.
Could you please describe what is meant by quantum entanglement?
Quantum Entanglement is a phenomenon where particles, or groups of particles, have their properties intimately intertwined such that they cannot be described independently of one another, even when separated by great distances. It appears as a direct consequence of the abstract mathematical formalism used in quantum mechanics. However, trying to translate and make sense of this abstract formalism in the physical world that surrounds us yields some very counter-intuitive results.
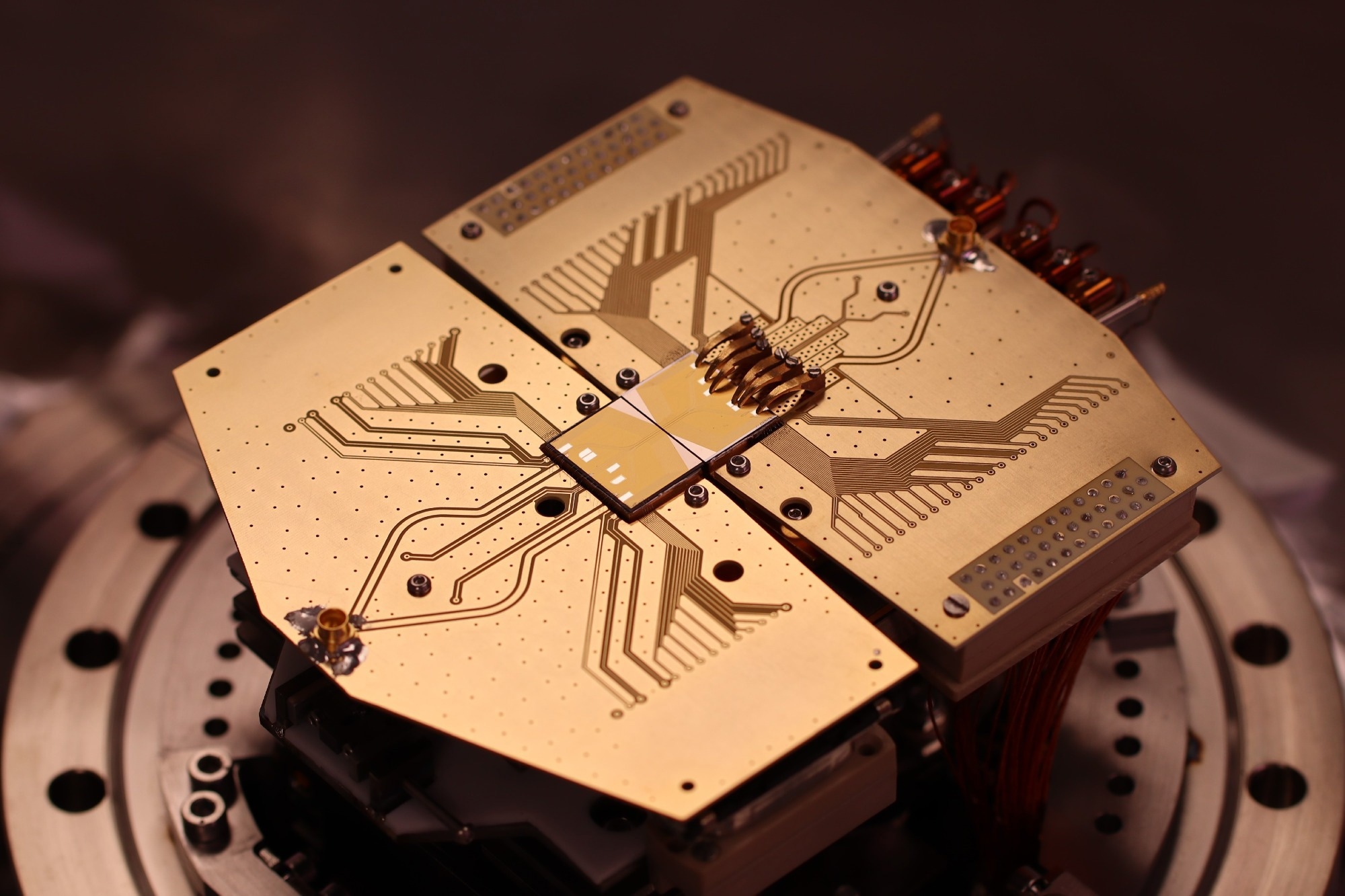
Most surprisingly, this extraordinary link between particles is predicted to give rise to an instantaneous interaction between them. This particularity is perhaps most famous for being pointed out by Einstein (in a 1935 paper commonly referred to as the EPR paradox after its authors, i.e., A. Einstein, B. Podolsky, and N. Rosen) who couldn’t quite wrap his head around it. Indeed, the phenomenon was so strange it appeared to defy some fundamental principles of our physical reality by seemingly transmitting information faster than the speed of light. He came to call it: “spooky action at a distance”.
Despite early doubts, this extraordinary phenomenon was demonstrated to be very real. In the 1980s, key experiments focused on producing and investigating the properties of entangled quantum states to an unprecedented degree eventually leading to last year’s Nobel Prize in Physics. Today, quantum entanglement is seen as one of the central characteristics of quantum mechanics.
Perhaps an illustration can help here. We can compare what happens between two imaginary quantum coins acting in place of our particles. As with our particles, coins have a set of properties. For instance, a coin being flipped can land “heads” or “tails”.
If our quantum coins are independent of one another and if we toss them at the same time, each will land giving a result that is fully uncorrelated from the other. There is no way of knowing on which side they will land, and we know that if the first coin lands “heads up,” this imparts no consequence on the side the second coin will land on.
Now, in the case of entangled quantum coins, each time the coins are tossed, the result will always be fully correlated. For instance, they can be entangled such that they will always land on opposite sides of one another, i.e., if the first coin lands “tails” the other coin will be landing “heads”. Even if flipped at the same time! Again, there is no way of knowing which side they will land on until flipped, but we can be certain that by looking at the result of one we will know that of the other. In this sense, we cannot think about our coins as two independent systems.
What role does quantum entanglement play in the functioning of quantum computers?
“Quantum entanglement” is one of the key phenomena alongside “quantum superposition” and “quantum interference” that are exploited by quantum computers to give significant computation speed-up when solving certain problems.
One formidable feature of entangled particles is that with every particle added, the number of quantum states that can be represented increases exponentially instead of linearly. In a quantum computer, each quantum state can be used to encode information. As a result, drastically fewer resources are required to store the same quantity of information on a quantum computer than on a classical computer.
Quantum computation is then carried out by carefully controlling the way entangled quantum states interfere with one another and themselves. This leads to either an increase or decrease in the probability associated with each quantum state. Quantum computation is then successful when the state associated with the correct output sees its probability of emerging out of the computational process amplified and reinforced.
What barriers to creating efficient quantum computers have existed in the past?
First, I should say that, while quantum computing is still in its infancy, it has come a significant way since it was first conceptualized in the early 1980s. Today, many researchers, in both academia and industry throughout the world, are capable of isolating, harnessing, and manipulating quantum systems with outstanding accuracy.
Quantum information can be encoded as quantum bits, or “qubits” in a variety of physical platforms, with amongst them “trapped ions” being a leading candidate. Indeed, quantum computers using trapped ions offer today some of the most promising attributes a quantum computer can seek: the quantum state of individual ion qubits can be manipulated with unrivaled accuracy while entangling operations used for quantum logic is executed with unmatched reliability.
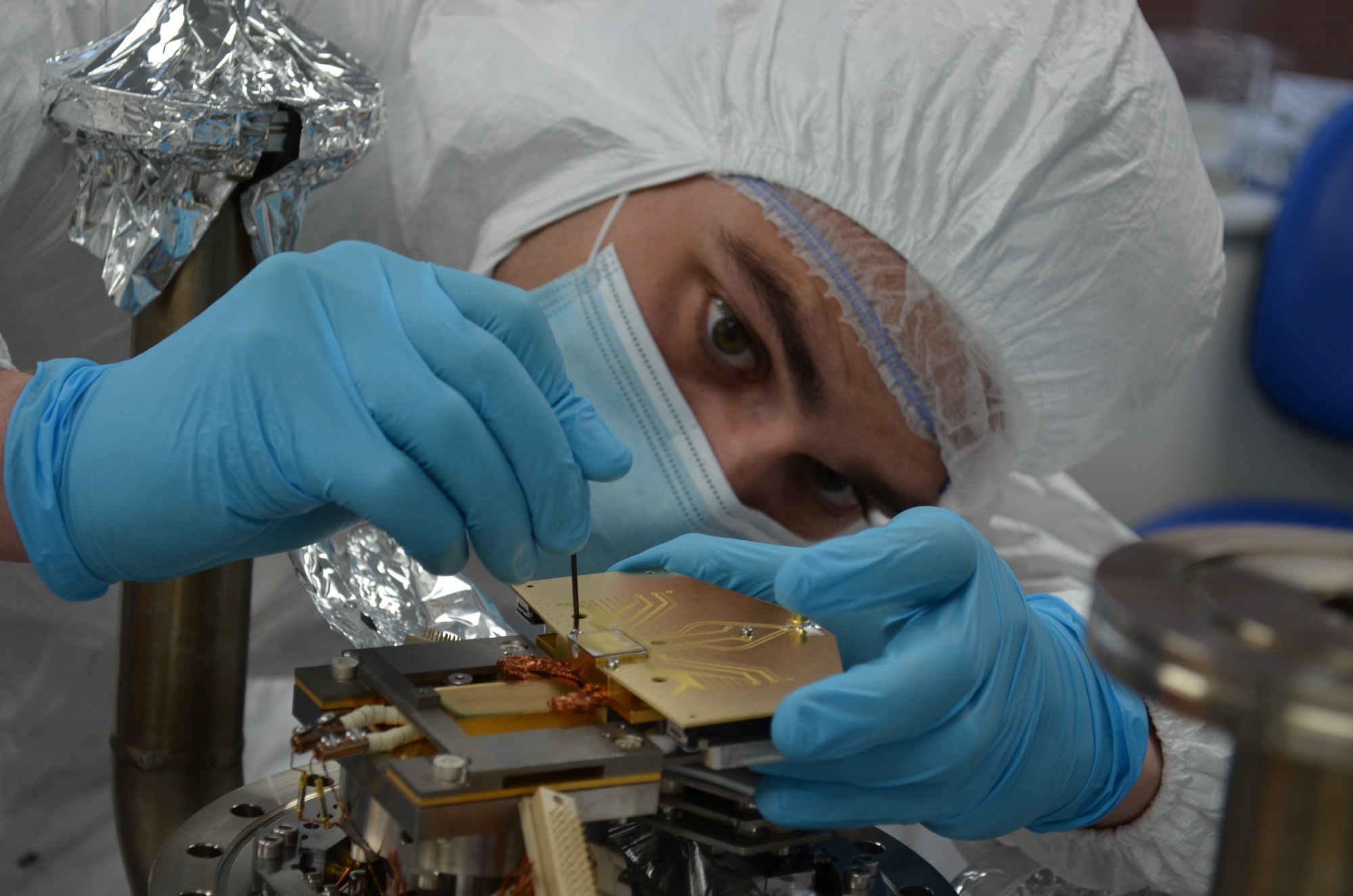
However, as discussed earlier, existing quantum computers are currently limited to hosting a handful of qubits, and this drastically hinders their ability to solve problems that have the potential to significantly impact people's lives.
In fact, most disruptive and anticipated quantum computing applications will require millions of qubits. This is because, despite being able to encode a large amount of information at the cost of few resources, quantum information remains extremely fragile and adversely interacts with its surrounding noisy environment. To further protect quantum computation from errors, a much larger number of qubits is then required to carry out a process known as quantum error correction such that small errors can be tolerated.
Some physical architectures have already been proposed and developed, to demonstrate key steps toward such fault-tolerant machines. Such architectures use ion-trap microchips and can be used to execute quantum algorithms via the simple application of voltages to an array of electrodes. But even then, the number of ion qubits that such a device can host remains limited by the size of the microchip.
Therefore, to overcome this obstacle, a utility-scale quantum computer must be modular and must also be provided with a means of connection between modules which ensures that the quantum information is transferred rapidly and reliably.
As a solution to this challenge, our research group theoretically proposed a few years ago to directly connect ion-trap quantum computer modules via an electric field and directly transport ion qubits between modules. However, the concept, which raised hopes for fast and high-quality inter-module connections, had until now never been demonstrated.
What is revolutionary about your latest research on quantum computing?
What our team from the University of Sussex and Universal Quantum has demonstrated is that the concept of connecting independent ion-trap quantum computer modules via electric fields is a viable method. Not only the method works, but it can be used for the inter-module transport of quantum information at high rates with minimal losses, thereby removing a key barrier towards scaling to utility-scale quantum computing machines.
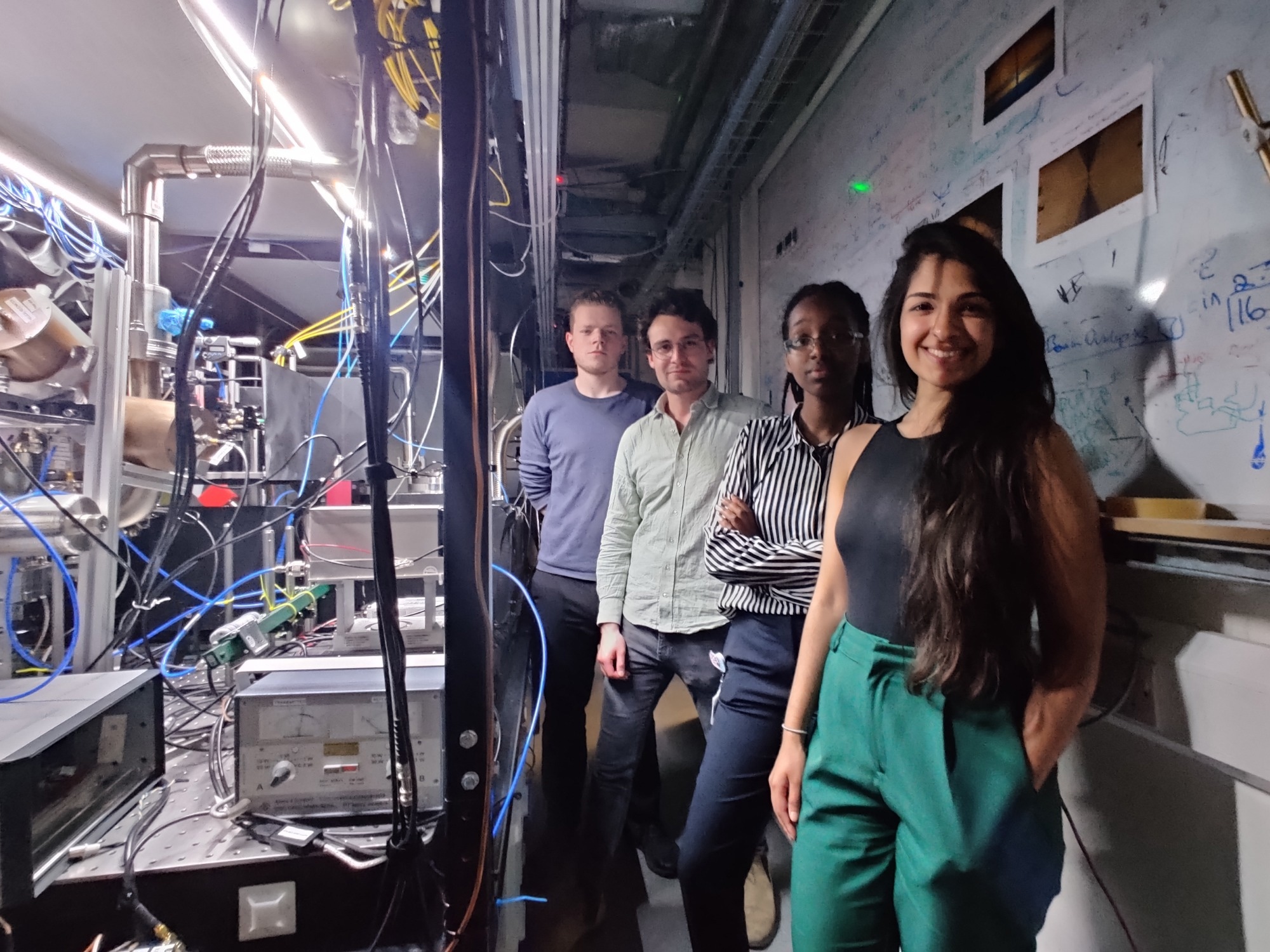
More specifically, in the experiment that was co-led between Falk Bonus, Mariam Akhtar, and myself, we demonstrated that trapped ions can be transferred at a record rate of up to 2,424 links per second. Throughout this operation, the ion has a probability of being lost of only 1 in 15 million, which is rather low! In addition, we’ve also demonstrated that the quantum information encoded within the trapped ion during a transport operation remains protected. These are all critical features for quantum computers to scale up.
How were you able to achieve such impressive reliability at record speeds?
To achieve these results, we have constructed a dedicated ion-trapping experiment featuring two ion-trap microchip modules housed in a vacuum apparatus.
These microchips consist of embedded micro-structured electrodes which span all the way to the modules' edges. By applying voltages to these electrodes, we are able to generate a tailored electric field that confines individual atomic ions above each module. In effect, we have individual ions that are trapped, levitating above the device's surface at a distance that is about the thickness of a human hair (here 125 microns).
The vacuum which surrounds the atoms is very high. In fact, the pressure in our vacuum apparatus is 100 trillion times less than the pressure exerted by the air that surrounds us. This ensures that the ion is left undisturbed, free of any collision with intruding background gases.
Each ion was then cooled using lasers through an effect known as Doppler cooling which brings its temperature down to the microkelvins range.
With the ion trap module closely aligned to within 10 microns, the electric fields generated on each module connected to one another and created a continuous confinement region above and in between both modules. Individual ions capable of carrying quantum information were then transferred from one module to the next by applying a specific sequence of voltages to the modules’ electrodes.
What still requires discovery or improvement before a quantum computing future is fully realized?
Now, it is true that this is one challenge removed with many still standing in the way. It will take time until we see a general-purpose quantum computing machine. But most of the remaining challenges are then of an engineering nature.
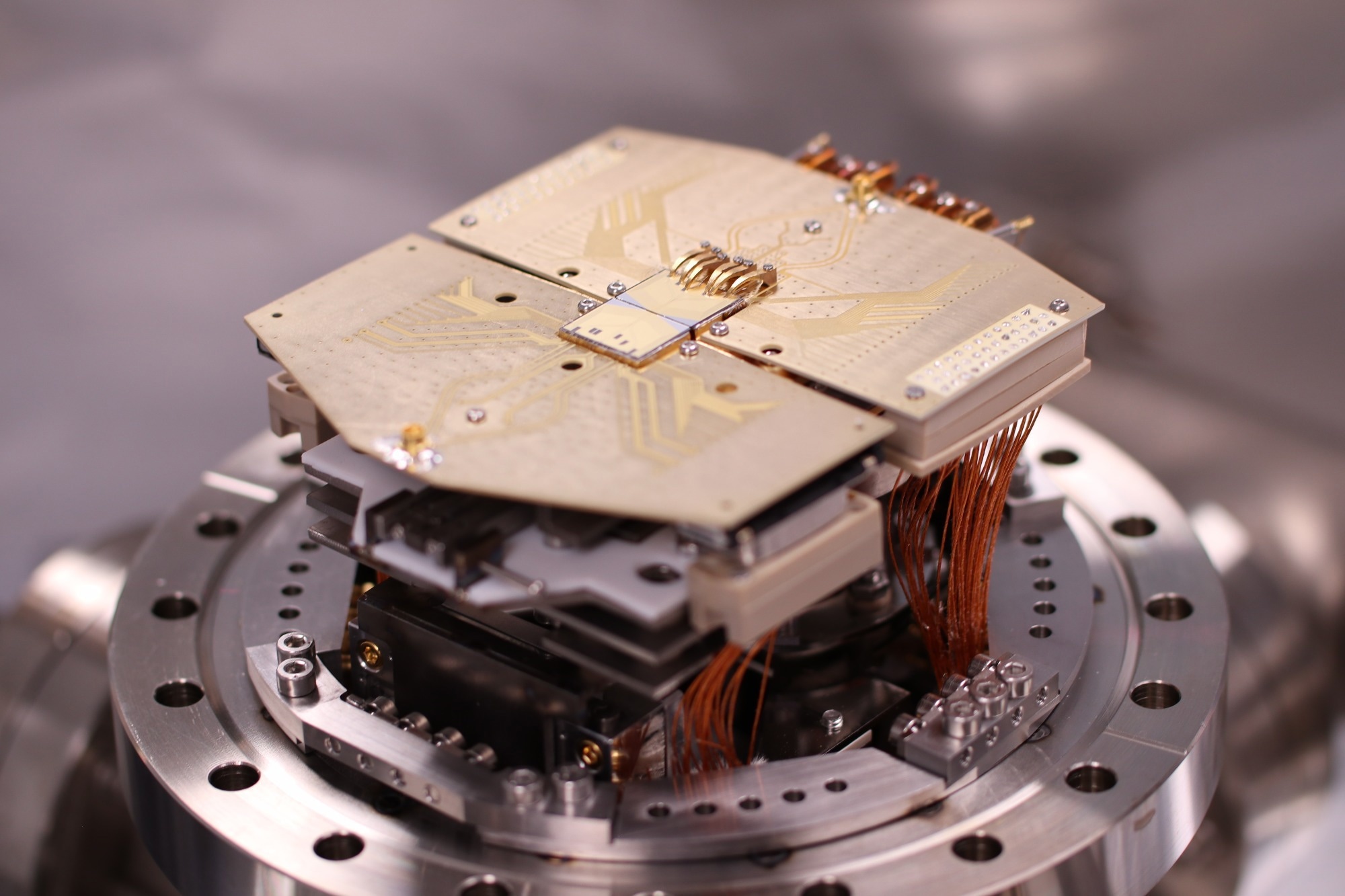
Now that the vast majority of ingredients needed for large-scale quantum computation have been demonstrated, it is all about bringing them together in one architecture. The undertaking of such an effort will be faced with a multitude of mutually conflicting requirements surrounded by extreme design and operating constraints. There are many hard challenges to solve. But they are not physically impossible ones, these are technically difficult ones that can be solved with the right resources, time, and expertise.
What kinds of applications could this technology be applied to in the future?
I should stress that a quantum computer is a scientific tool that will most likely find application in R&D development and will be used to solve a very specific set of problems. It will first be an enabling technology. A quantum computer is not a silver bullet capable of healing all ailments! This is because a quantum computer is very well suited to solve certain classes of problems. It's not about performing the same classical computation faster, it's about finding computational problems, which can be effectively solved in an overall fewer amount of steps when using the rules of quantum mechanics.
We’re hoping that by making use of the technology that we have demonstrated that quantum computers will scale to qubit numbers sufficiently large for them to start solving meaningful problems. By this, I mean advancing a variety of fields that have a tangible effect on the lives of people, such as by helping create new materials and developing new drugs, but also assisting society to become more sustainable.
What is personally the most exciting aspect of this research to you?
While it is true that this is one challenge removed with many still standing in the way, what makes me personally most excited is that we've removed the uncertainty of whether it is physically possible or not to scale up trapped ion quantum computers.
But that change alone in the nature of the problem is an entire paradigm shift in the way we approach the development of quantum computers. And that is fantastic because both at Sussex and Universal Quantum we have a clear engineering roadmap for constructing a practical quantum computing machine using this technology.
What are the immediate next steps?
We are already working on an upgrade! The system is currently being fitted with improved, high-performance electronic hardware such that we can push these connection rates even faster. In addition, we are already working closely with the rest of the engineering teams at Universal Quantum to deploy this technology as part of the multi-module quantum computer that is being constructed for the German Aerospace Center (DLR) in Hamburg.
One of the other immediate next steps is also that we are hiring! So, if you are a researcher, engineer, or technician and would love to be a part of this project or work on another exciting quantum tech challenge in academia or industry, please come join us!
Where can readers find more information?
Research article:
https://www.nature.com/articles/s41467-022-35285-3
Research group (IQT) and University spin-out (Universal Quantum) webpage:
https://www.sussex.ac.uk/physics/iqt/
https://universalquantum.com/
Other News articles:
https://www.bbc.co.uk/news/science-environment-64492456
https://medium.com/@universalquantum/record-breaking-discovery-solves-major-quantum-puzzle-by-fitting-computers-together-like-a-2b330bd92865
https://physicsworld.com/a/uk-firm-universal-quantum-wins-major-quantum-computing-contract
About Dr. Foni Raphaël Lebrun-Gallagher
I am presently a Research Fellow in Trapped Ion Quantum Computing at the Sussex Centre for Quantum Technology at the University of Sussex and a Quantum Advisor for Sussex's university spin-out quantum computing company Universal Quantum. I am currently working in the research group headed by Prof. Winfried Hensinger. My work focuses on developing scalable hardware solutions to overcome the outstanding physics and engineering obstacles that stand on the way of making quantum computers practical. I hold an undergraduate degree in Physical Sciences from the University of Rennes 1 (France). I obtained my MSc in Quantum Technology at the University of Sussex (Department of Physics and Astronomy) and a PhD in Trapped Ion Quantum Computing also at the University of Sussex (Ion Quantum Technology group).
Disclaimer: The views expressed here are those of the interviewee and do not necessarily represent the views of AZoM.com Limited (T/A) AZoNetwork, the owner and operator of this website. This disclaimer forms part of the Terms and Conditions of use of this website.