Black holes are cosmic phenomena with immense gravitational pull, but scientists have been unable to study them thoroughly, as recreating such extreme environments in a laboratory setting has long been a challenge. However, a recent study has introduced a novel optical black hole device that mimics their behavior, offering a new pathway for experimental investigation.
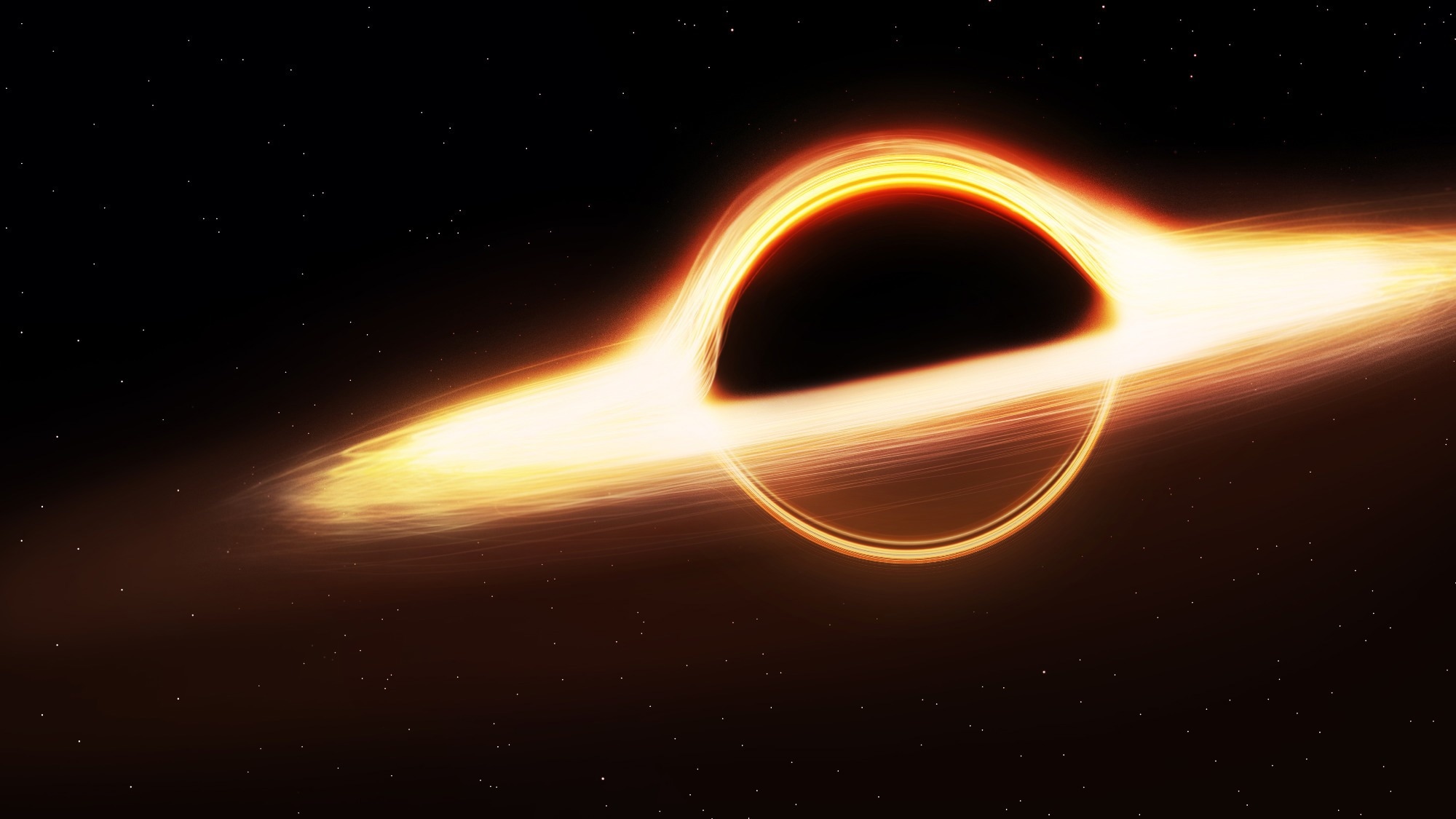
Image Credit: Lia Koltyrina/Shutterstock.com
The optical black hole device operates on the principles of coherent perfect absorption, enabling controlled manipulation of light based on its polarization state. This innovative approach offers a novel experimental platform for exploring complex electromagnetic phenomena, providing deeper insights into fundamental physics.
Additionally, it opens new possibilities for practical applications in energy harvesting, sensing, and communication, marking a significant advancement in quantum physics, optics, and photonics.1
The Science Behind It
A black hole is a spacetime region where gravitational forces become so intense that neither matter nor electromagnetic radiation can escape. It is formed when a massive star collapses under its gravity after exhausting its nuclear fuel, resulting in a singularity of infinite density. Its most defining feature is the event horizon, the boundary beyond which nothing can escape, as the intense gravitational pull warps spacetime to an extreme degree.2
Optical analogs help replicate these astrophysical phenomena in laboratory environments using metamaterials and nanostructured media to introduce spatially varying refractive indices. These engineered media guide light in ways that emulate photon trajectories in strong gravitational fields, reproducing general relativistic effects such as light bending and unidirectional radiation flow near an event horizon.
Unlike actual black holes, which require extreme mass and gravity, optical analogs achieve similar behavior through coherent electromagnetic waves in subwavelength architectures, enabling laboratory investigations of relativistic and quantum effects beyond the reach of direct astronomical observation.3
No time to read now? Download your PDF copy here!
How does the Black Hole Device Work?
The optical black hole device proposed in Advanced Photonics leverages advanced optical principles to replicate the behaviors of black holes. It comprises two identical 90-degree BK7 glass prisms separated by a 20-nanometer chromium absorber layer.
Unlike prior optical black hole analogs that rely on metamaterials to simulate spacetime curvature via spatial refractive index gradients, this system uses a simplified mechanism based on coherent perfect absorption and transmission. It manipulates electromagnetic waves through interference rather than gravitational effects; spatially coherent light entering the device generates counterpropagating beams that interfere at the thin absorber interface.
The polarization state of this light determines the interference outcome: s-polarized light undergoes constructive interference and is nearly fully absorbed, mimicking black hole behavior, whereas p-polarized light undergoes destructive interference and is mostly transmitted, replicating white hole behavior.
The rapid angular transition between these states near normal incidence constitutes an optical analog to the event horizon, providing a compact and accessible platform for examining relativistic phenomena in laboratory settings.1
The Tech and Innovation
Recent advancements in metamaterials, nanophotonics, and thin-film engineering have been pivotal in developing the optical black hole device.
Shraddha M. Rao et al., in Optical Letters, demonstrated all-optical modulation in multilayered graphene films with up to 80% efficiency, utilizing a standing wave created by counter-propagating laser beams. Their research showcased how the position of a subwavelength film within an interference field can precisely control the modulation of scattered energy.4
Similarly, Jianfa Zhang et al., in Light: Science & Applications, revealed that coherent interactions between low-intensity light beams on nanoscale metamaterial layers could either eliminate plasmonic Joule losses or induce near-total absorption, facilitating ultrafast all-optical pulse recovery and terahertz-bandwidth modulators. These innovations paved the way for creating subwavelength absorbers capable of precisely manipulating electromagnetic waves.5
Additionally, computational modeling tools such as COMSOL Multiphysics were integral in simulating the device's electromagnetic field behavior. The simulations provided insights into how traveling waves propagate for the black hole case and how standing waves form for the white hole analog, replicating gravitational effects within an optical setup.1
Potential Applications
The optical black hole device presents a range of applications in both fundamental physics and applied engineering.
Analog Platform for Gravitational Physics
The optical black hole device serves as a laboratory-scale analog for gravitational systems, enabling precise investigation of electromagnetic wave behavior under conditions that emulate spacetime curvature near black holes. This controlled setup allows physicists to test theoretical models related to general relativity and light-matter interactions, bypassing the need for astrophysical environments.
Energy Harvesting and Electromagnetic Control
The device's polarization-selective absorption and transmission properties facilitate advanced electromagnetic wave manipulation, enabling efficient broadband energy harvesting, multispectral camouflage, and stealth technologies. By achieving complete absorption of targeted polarizations, it optimizes energy capture across a broad spectral range, enhancing performance in systems that require tailored electromagnetic responses.
Compact Photonic Integration for Light Routing and Sensing
Deterministic control of light propagation enables the development of compact photonic components for trapping, sensing, and optical communication. In addition, the device's planar geometry and subwavelength thickness facilitate integration into photonic systems, where polarization-sensitive control provides additional functionality for signal routing and optical processing.
Broadband Optical Platform for Nonlinear and Metamaterial Research
As a model system for extreme light-matter interactions, the device enables the investigation of nonlinear optical effects, energy concentration mechanisms, and absorption phenomena. These insights advance the development of metamaterials and engineered photonic media. Its broadband operational range supports applications across quantum optics, thermal management, and radiation control, where spectral flexibility is essential.1,6
Conclusion
The optical black hole device represents a significant innovation, replicating the functional behavior of a black hole through finely tuned optical properties without requiring spacetime warping. This breakthrough provides a unique framework for investigating light behavior under extreme conditions, offering deeper theoretical and practical insights into electromagnetic field control.
As nanofabrication and metamaterial science evolve, such optical analogs will play a key role in shaping future photonic technologies, enabling unprecedented control over light and mimicking gravitational effects.
References and Further Reading
- Plum, E., Vetlugin, A. N., Salimzhanov, B., Zheludev, N. I., & Vaidya, N. (2025). Optical analog of black and white gravitational holes. Advanced Photonics, 7(2), 025001-025001. https://doi.org/10.1117/1.AP.7.2.025001
- Francis Reddy. (2020). What Are Black Holes? [Online]. https://www.nasa.gov/universe/what-are-black-holes/
- Narimanov, E. E., & Kildishev, A. V. (2009). Optical black hole: Broadband omnidirectional light absorber. Applied Physics Letters, 95(4). https://doi.org/10.1063/1.3184594
- Rao, S. M., Heitz, J. J., Roger, T., Westerberg, N., & Faccio, D. (2014). Coherent control of light interaction with graphene. Optics letters, 39(18), 5345-5347. https://doi.org/10.1364/OL.39.005345
- Zhang, J., MacDonald, K. F., & Zheludev, N. I. (2012). Controlling light-with-light without nonlinearity. Light: Science & Applications, 1(7), e18. https://doi.org/10.1038/lsa.2012.18
- Daneet Steffens. (2025). Scientists create optical device that mimics black holes. [Online]. https://www.eurekalert.org/news-releases/1080571
Disclaimer: The views expressed here are those of the author expressed in their private capacity and do not necessarily represent the views of AZoM.com Limited T/A AZoNetwork the owner and operator of this website. This disclaimer forms part of the Terms and conditions of use of this website.