Particles in quantum mechanics can exist in more than one state simultaneously, defying common sense.
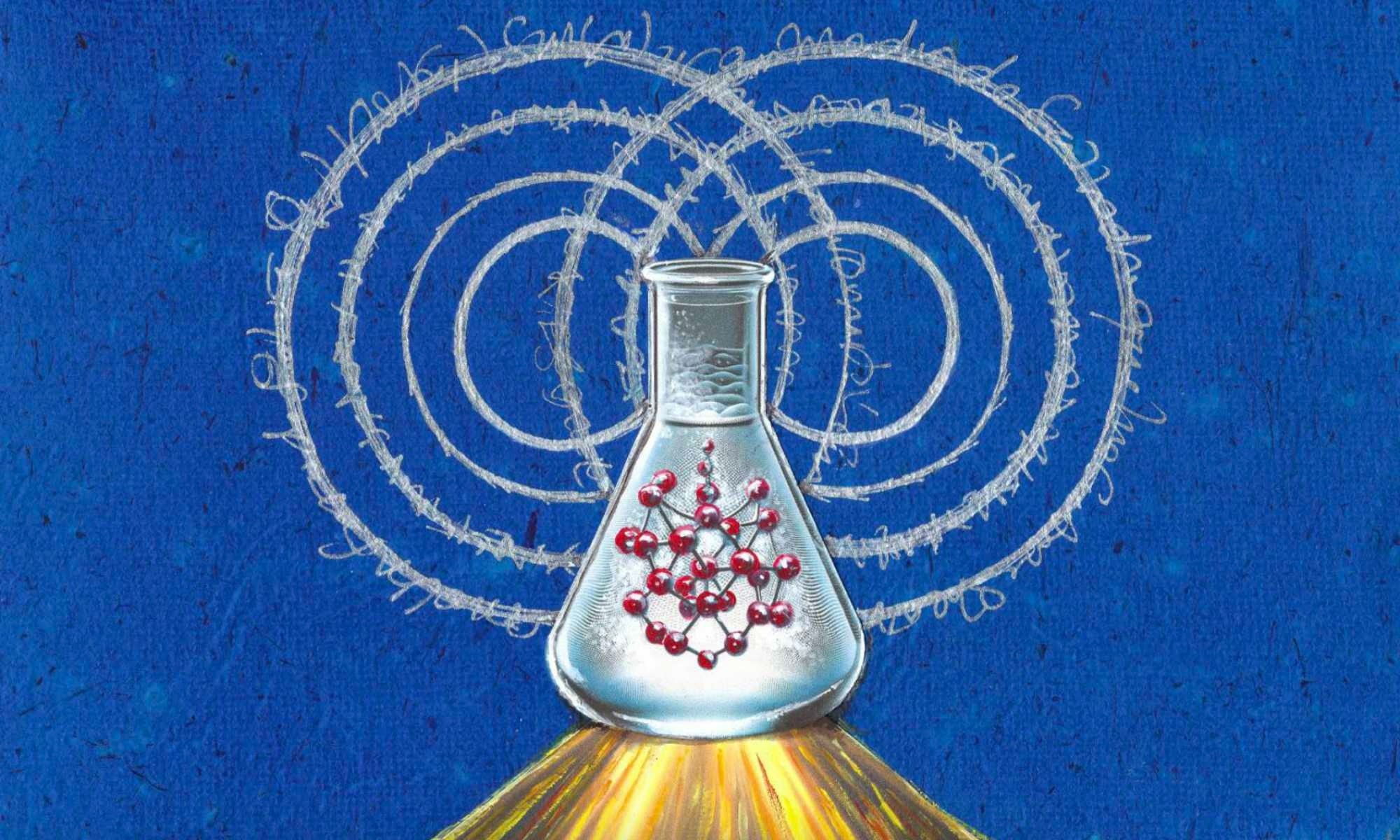
Rochester researchers have reported a strategy to understand how quantum coherence is lost for molecules in solvents with full chemical complexity. The findings open the door to the rational modulation of quantum coherence via chemical design and functionalization. Image Credit: Anny Ostau De Lafont
Emerging quantum technologies, or quantum superposition, are based on this property and have the potential to revolutionize sensing, computing, and communication. However, quantum decoherence poses a serious obstacle to quantum superpositions. When the delicate superposition of quantum states interacts with its surroundings during this process, it collapses.
Scientists must comprehend and manage quantum decoherence to create molecules with particular quantum coherence characteristics, which will allow them to harness the power of chemistry to create intricate molecular architectures for useful quantum applications. To do this, one must be able to rationally alter the chemical structure of a molecule in order to reduce or modulate quantum decoherence. To achieve this, researchers must determine the “spectral density,” a measurement that encapsulates the rate of entanglement and strength of the environment with the quantum system.
Until now, theory and experiment have not been able to quantify this spectral density in a way that truly captures the complexities of molecules. However, a group of researchers has created a technique that uses straightforward resonance Raman experiments to extract the spectral density for molecules in a solvent, capturing the entire intricacy of chemical environments. The group, led by University of Rochester Associate Professor of Physics and Chemistry Ignacio Franco, published the results in the Proceedings of the National Academy of Sciences.
By utilizing the extracted spectral density, one can ascertain the primary contributor to the decoherence as well as its rate of occurrence within the chemical environment. Thus, decoherence pathways can now be mapped by scientists to establish a connection between quantum decoherence and molecular structure.
Chemistry builds up from the idea that molecular structure determines the chemical and physical properties of matter. This principle guides the modern design of molecules for medicine, agriculture, and energy applications. Using this strategy, we can finally start to develop chemical design principles for emerging quantum technologies.
Ignacio Gustin, First Author and Graduate Student, Department of Chemistry, University of Rochester
The breakthrough occurred when the team realized that all the information required to study decoherence with full chemical complexity could be obtained from resonance Raman experiments. Although these experiments are frequently used to study photochemistry and photophysics, the experiment’s usefulness for quantum decoherence has not been recognized.
The main insights came from conversations with two experts, one of whom was a postdoctoral researcher at Rochester and is now an Associate Professor in the department of chemistry there; the other is Chang Woo Kim, who is an expert in quantum decoherence and is currently on the faculty at Chonnam National University in Korea.
The team utilized their method to unveil a groundbreaking revelation: the rapid unraveling of electronic superpositions in thymine, a fundamental DNA component, occurs within a mere 30 femtoseconds (one femtosecond equates to one-millionth of one billionth of a second) subsequent to absorbing UV light.
Their study highlighted the influence of specific molecule vibrations on the initial phases of the decoherence process, while the solvent plays a dominant role in the subsequent stages. Furthermore, their findings showcased that modifications to thymine can significantly impact the decoherence rate, particularly emphasizing that hydrogen-bond interactions near the thymine ring accelerate this process.
The group’s work paves the path for comprehending the chemical laws governing quantum decoherence.
We are excited to use this strategy to finally understand quantum decoherence in molecules with full chemical complexity and use it to develop molecules with robust coherence properties.
Ignacio Franco, Department of Chemistry, University of Rochester
Journal Reference
Gustin, I., et al. (2023). Mapping electronic decoherence pathways in molecules. PNAS. /doi/10.1073/pnas.2309987120