Oct 23 2019
A team of researchers from Cornell University has applied a new twist to measuring and manipulating spins in nickel oxide, with a keen interest toward enhancing the memory capacity and speed of electronic devices.
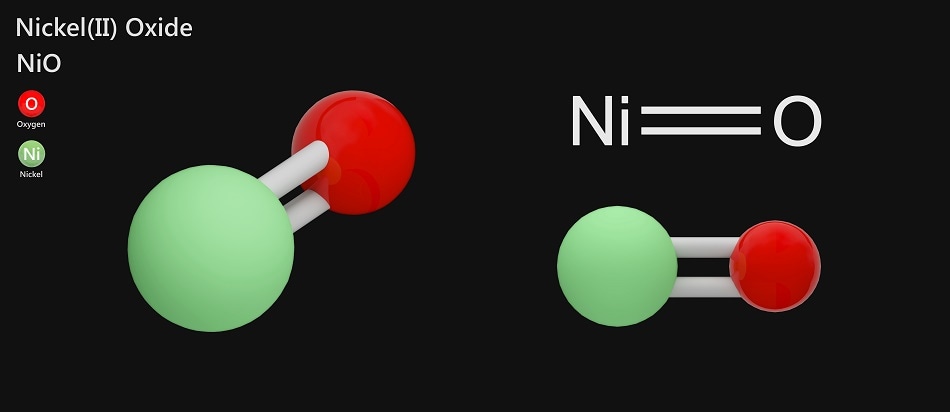
Image Credits: Orange Deer Studio/shutterstock.com
Their paper titled “Spin Seebeck Imaging of Spin-Torque Switching in Antiferromagnetic Pt/NiO Heterostructures” has been published in the October 22nd issue of the Physical Review X.
One of the areas of expertise of senior author Greg Fuchs, associate professor of applied and engineering physics, is spintronics — the analysis of the spin (a kind of angular momentum that can be utilized to record information) that governs the magnetic properties of electrons. Fuchs and his team are determined to understand how to measure and control this magnetism.
Instead of measuring magnetism with conventional forms of magnetic microscopy — wherein materials are struck with electrons, light, or X-rays — Fuchs has mastered a technique known as magneto-thermal microscopy. This method involves applying heat to a small area of the material, and evaluating magnetism in that area by measuring the electrical voltage that is produced. Thus, Fuchs and his team were able to observe what takes place when the spins of a magnetic material are controlled.
The Fuchs Group has been investigating antiferromagnetic materials, which are distinctive because their individual magnetic elements—the minute pieces of material that recall bits of information based on their orientation — do not yield a magnetic field. Thus, they can be packed tightly together without unsettling each other, potentially allowing high-density storage.
Antiferromagnets are even faster relatives of ferromagnets—more conventional magnetic materials that do create a magnetic moment. According to Fuchs, antiferromagnets have the ability to work a thousand times faster.
However, it is not easy to gain insights into the behavior of antiferromagnetic materials.
Antiferromagnetic material is hard to study because every other spin points in the opposite direction, so there is no net magnetization. It doesn’t create a magnetic field. It’s not really amenable to conventional approaches to magnetic measurement. There are specialized X-ray facilities that can do it, but there aren’t many, and that limits the measurements that you can make. So you have very few options.
Greg Fuchs, Study Senior Author and Associate Professor of Applied and Engineering Physics, Cornell University
Fuchs and his colleagues developed a smart end-run around the problem by selecting just the right kind of antiferromagnetic material — nickel oxide — which has several planes of spin, where the spins in every other plane face in an opposite direction. In a sandwich of nickel oxide and platinum, the spins at the boundary are all arranged parallel to one another, enabling scientists to use heat flow to measure the orientation of the spins without canceling out the signal.
This effect, known as the “interfacial spin Seebeck effect,” had been demonstrated earlier in ferromagnetic metals and insulators, but was only speculated for antiferromagnets. Thus far, researchers have not demonstrated it — let alone attempt to use it to image antiferromagnetic samples with regular tabletop lab equipment.
Imaging antiferromagnets allows us to see microscopically how they respond to external stimuli, such as electrical current. These details are critical when trying to make antiferromagnetic memory devices.
Isaiah Gray, Study Lead Author and PhD Student in Applied Physics, Cornell University
“You normally think of antiferromagnets as a pretty hard nut. It’s surprising to me that such a relatively simple approach works,” stated Fuchs. “This unlocks a whole new realm in terms of what you can do in antiferromagnetic devices. Now I can control the textures in these materials, and then see how the spins are oriented.”
The co-authors of the study are assistant professor Katja Nowack; Daniel Ralph, the F.R. Newman Professor of Physics; Darrell Schlom, the Herbert Fisk Johnson Professor of Industrial Chemistry; postdoctoral associates Nikhil Sivadas, Gregory Stiehl, and John T. Heron; applied physics PhD student David Low; and scientists from Kyoto University, the University of Michigan, and the National Institute of Standards and Technology Center for Neutron Research.
The study received support from the Cornell Center for Materials Research, with financial assistance from the National Science Foundation’s Materials Research Science and Engineering Center program, and the Japan Society for the Promotion of Science.