Many atomic nuclei possess a magnetic field similar to that of the Earth. However, at the surface of a heavy nucleus, such as lead or bismuth, it is trillions of times stronger than the Earth’s field and more similar to that of a neutron star.
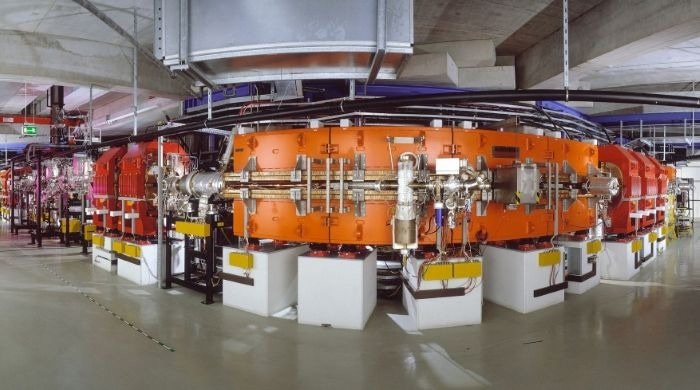
In the experimental storage ring ESR, the ions were stored at about 72% of the speed of light and bombarded with laser pulses traveling in opposite directions. Image Credit: Mai, GSI/FAIR
According to a study published in Nature Physics, a TU Darmstadt-led research team at the GSI Helmholtz Centre for Heavy Ion Research has taken a significant step in understand the behavior of an electron in such powerful fields. The results support the theoretical predictions.
Hydrogen-like ions, or atomic nuclei with only one electron, are theoretically very simple to characterize. In the case of heavy nuclei with a high proton number, such as bismuth, which has 83 positively charged protons in its nucleus, the strong electrical attraction attaches the electron close to the nucleus, bringing it within this intense magnetic field.
The electron aligns its magnetic field with the nucleus’s field, much like a compass needle. With just the right amount of energy, this "needle" can be flipped in the opposite direction. That’s precisely what the researchers accomplished. They successfully applied this technique to a radioactive isotope, guided by a highly accurate theoretical prediction of the exact energy required.
The required energy can be determined using quantum electrodynamics (QED), which is the quantum theory of electromagnetism. However, a lack of understanding of the structure of such heavy atomic nuclei makes accurate predictions impossible and has thus far prevented a precise and unambiguous test of the hypothesis. Measurements of the stable isotope Bi-209 have confirmed the theoretical prediction.
However, there were still concerns about whether the influence of the nuclear structure on the theoretical prediction could be minimized to the amount claimed. To overcome this gap in the experimental test, it was recommended to analyze another bismuth isotope with a different nuclear structure.
Since bismuth does not have a second stable isotope, thus the research team led by Professor Wilfried Nörtershäuser had to use a radioactive isotope. The isotope Bi-208 was a good option since it had one less neutron than the stable isotope, resulting in an even greater magnetic field.
Magnetic Field of the Electron is Flipped
The initial challenge in this experiment was to generate and isolate the hydrogen-like ion of the desired isotope Bi-208.
Dr. Max Horst, Study Lead Author, TU Darmstadt
To do this, a neutron was pushed out of the stable Bi-209 via a nuclear reaction, and the resulting fragments were collected in the ESR experimental storage ring. At the same time, all but one of the atom’s initial 83 electrons must be taken away to create the hydrogen-like configuration.
The fragments circle the storage ring at roughly 72% of the speed of light or approximately 200,000 kilometers per second. The hydrogen-like ions of the isotope Bi-208 were discovered and all undesirable reaction products were eliminated.
Horst added, “In earlier measurements of the stable isotope Bi-209, we had around 1,000 times more ions available which is why all aspects of the experiment had to be optimized in terms of efficiency and sensitivity.”
The measurement principle is based on irradiating the electron’s magnetic field with a laser beam of the appropriate energy. The ion absorbs an elementary particle of light, known as a photon, from the laser beam. The photon’s energy is subsequently transmitted to the electron and used to flip its magnetic field, resulting in the unfavorable alignment of the nuclear magnetic field.
To release this energy, the electron flips back after roughly a half-millisecond on average, producing another photon. By this point, the ion has circled the storage ring hundreds of times, and the released photons are detected by sensitive detectors in a particularly dark spot along the storage ring to minimize background noise.
Accurate Prediction was Crucial
Given the limited quantity of ions, it was essential to make a very precise prediction regarding the photon energy or laser wavelength (or “color”) at which the process should occur. Nörtershäuser points out that it would have required a lot of time to search a wide wavelength range at such a low signal rate.
He thus started measuring the neutral atoms of the two Bi isotopes at the CERN research center in Switzerland a few years ago, enabling the estimation of the impact of the various nuclear configurations.
By combining this data with the previous measurement of the stable isotope's hydrogen-like ion, the theoretical physicists were able to estimate the transition energy in hydrogen-like Bi-208 with extreme precision. This value was around ten times more exact than a full quantum mechanical calculation, but it still agreed with it. This prediction is very well supported by the experimental value that was observed at the storage ring and reported in the new study.
The results can now be used to forecast the effect of the nuclear structure on other charge states of the isotope Bi-208, and the process can be applied similarly to other bismuth isotopes or other elements.
The findings are largely the result of a collaboration between TU Darmstadt, researchers at the GSI Helmholtz Centre for Heavy Ion Research in Darmstadt, and the Universities of Münster and Jena, which was supported by the German Federal Ministry of Education and Research (BMBF) as part of the APPA Research Focus. Furthermore, the Helmholtz Research Academy Hesse funded the scientific efforts of TU Darmstadt, the GSI Helmholtz Centre, and Justus Liebig University Giessen.
Journal Reference:
Horst, M. et al. (2025) Storage-ring laser spectroscopy of accelerator-produced hydrogen-like 208Bi82+. Nature Physics. doi.org/10.1038/s41567-025-02885-x