In a paper published in the journal Nature Communications, researchers explored Bell nonlocality, challenging classical causality with correlations between distant, entangled particles. They showed that single copies of Bell-local states could exhibit nonlocality within a quantum network. The authors certified nonlocality without assumptions by subjecting an initial entangled state to a quantum channel. These findings advance the understanding of nonlocality and its potential applications.
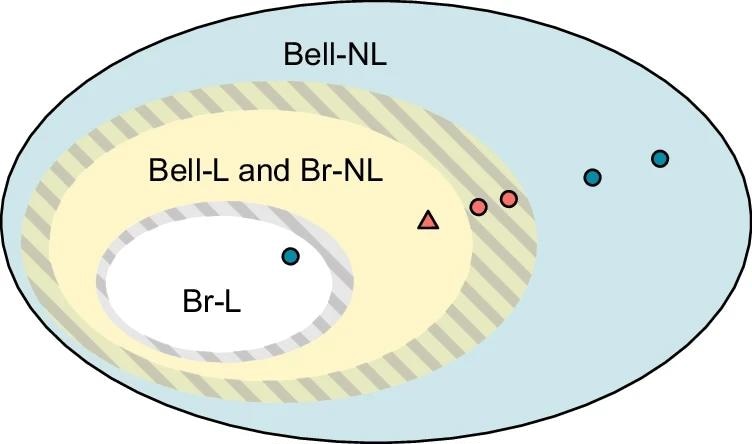
The activation of nonlocality is certified for any experimental state that is rigorously proven to belong to the set producing both Bell-local and broadcast-nonlocal correlations, as illustrated by the triangle symbol. Striped areas represent uncertainties for determining the boundaries between correlation sets. Image Credit: https://www.nature.com/articles/s41467-024-47354-w
Modeling Broadcast Scenario: Overview and Analysis
In this study, the researchers outlined their approach to modeling the broadcast scenario, considering classical and quantum descriptions. They introduced a formalism to assess the joint probability distribution resulting from the application of a broadcast channel on a given state, emphasizing the importance of relaxing constraints on the channel to allow for various resources. Additionally, the no-signaling condition between the broadcast parties was formalized, highlighting its role in the experimental setup's causal structure.
The team developed an algorithm building upon previous frameworks to certify local hidden variable (LHV) models for quantum states, utilizing positive maps and known LHV models for specific states. They efficiently derived LHV models for experimental states through computational methods, facilitating their analysis of nonlocality activation within the broadcast scenario.
The experimental setup focused on photon sources and the implementation of the broadcast channel. The researchers detailed the generation of single photons using type-II spontaneous parametric down-conversion (SPDC), highlighting the controlled depolarizing channel's role in preparing experimental states akin to two-qubit isotropic states.
The study underscored the meticulous calibration and characterization required for quantum state tomography, which is crucial for preventing the mischaracterization of experimental states in subsequent tests. In addition, the team described their instance of a suitable broadcast channel, inspired by previous work, and its implementation using single-qubit rotations and controlled-negation (NOT) gates.
The study clarified the protocol for projective measurements, which includes the binary variable for determining each party's measurement choices and the setup for polarization experiments. The authors described how they detected photons using superconducting nanowire single-photon detectors (SNSPDs) and collected coincidence events over a long period. They stressed the importance of carefully choosing measurement settings to maximize the violation of the specified inequality using the broadcast channel they implemented.
The study discussed the experimental error analysis, including uncertainties in state parameters and locality certificates derived from tomographic reconstructions that account for both systematic and statistical errors. It also highlighted the distinction between device-dependent tasks, such as quantum state tomography, and device-independent tasks, like evaluating broadcast inequality. Additionally, the study presented a method for calculating uncertainties in inequality values, emphasizing the importance of photon counting statistics and error propagation techniques.
Overall, the study offered a comprehensive overview of the researchers' theoretical framework, experimental setup, and error analysis, elucidating the intricacies of their approach to studying nonlocality activation within the broadcast scenario.
Exploring Nonlocality in Quantum Networks
The researchers compared the traditional Bell scenario, which tests nonlocality by analyzing correlations between measurement outcomes to see if they adhere to a classical causal structure, with their three-node quantum network setup. Violations of Bell inequalities in this context are indicative of nonlocality.
In their network, an additional channel modifies part of the initial state, distributing information to further parties. The researchers developed a formalism to evaluate correlations in this scenario, emphasizing the need to relax channel constraints to accommodate various resources. By enforcing no-signaling conditions between parties, they focused on detecting nonlocality that originates solely from the initial source.
The experimental objective was to demonstrate nonlocality by revealing correlations that challenge classical descriptions. The researchers sought to confirm that their bipartite states could not be explained locally in standard Bell scenarios. To facilitate this, they introduced an algorithm designed to build local hidden variable models for experimental states, specifically tailored for general two-outcome measurements. This algorithm utilized established models for specific states to efficiently analyze the experimental ones. Additionally, their photonic setup, featuring high-quality photon sources and a controlled broadcast channel, was key in rigorously testing the activation of nonlocality.
Experimental results demonstrated significant violations of classical limits, signaling the activation of nonlocality for certain states. Careful evaluations of the Bell locality of these experimental states underscored the critical need for rigorous analysis, cautioning against premature assumptions regarding their conformity to expected models. Initial assessments suggested Bell locality based on state parameters, but detailed testing later exposed more nuanced outcomes, highlighting the essential role of comprehensive locality analysis.
This research enhances our understanding of nonlocal correlations in quantum networks, demonstrating the potential to access nonlocality beyond conventional noise constraints. By integrating an intermediate quantum hub, the study showed how nonlocality can be realized in more complex network configurations. These results set a foundation for future developments in quantum communication and information processing. Emphasizing the importance of hybrid assumptions in network scenarios, the findings are crucial for accurately characterizing correlations in increasingly sophisticated systems.
Conclusion
To sum up, the researchers embarked on a journey to explore nonlocality within quantum networks, comparing it to traditional Bell scenarios. They developed a formalism to assess correlations in a three-node setup, aiming to identify nonlocality originating from the initial source alone.
Through detailed experimental testing, they successfully demonstrated nonlocality, revealing violations of classical limits and confirming that their bipartite states could not conform to standard Bell scenarios. This study deepens the understanding of nonlocal correlations and sets the stage for future advancements in quantum communication and information processing, especially within complex network scenarios.
Journal Reference
Villegas-Aguilar, L., et al., (2024). Nonlocality activation in a photonic quantum network. Nature Communications, 15:1, 3112. https://doi.org/10.1038/s41467-024-47354-w, https://www.nature.com/articles/s41467-024-47354-w.
Disclaimer: The views expressed here are those of the author expressed in their private capacity and do not necessarily represent the views of AZoM.com Limited T/A AZoNetwork the owner and operator of this website. This disclaimer forms part of the Terms and conditions of use of this website.
Article Revisions
- Apr 17 2024 - Title changed from "Nonlocality in Quantum Networks: From Theory to Experiment " to "Exploring Nonlocality in Quantum Networks"
- Apr 17 2024 - Revisions made to certain sentences to improve grammar.