Hematite (Fe₂O₃) is a common iron mineral found in abundance on Earth, and analyses of surface samples have also confirmed its presence on Mars. Hematite plays a key role in the redox cycling of iron and is well-known as a cost-effective semiconductor with the ability to absorb a significant portion of visible light.1 Hematite is primarily used in the production of steel and iron, but its role as a pigment stands out as one of its most economically significant applications.2 In recent years, a novel trend has emerged focusing on the use of Hematite in next-generation computational platforms.
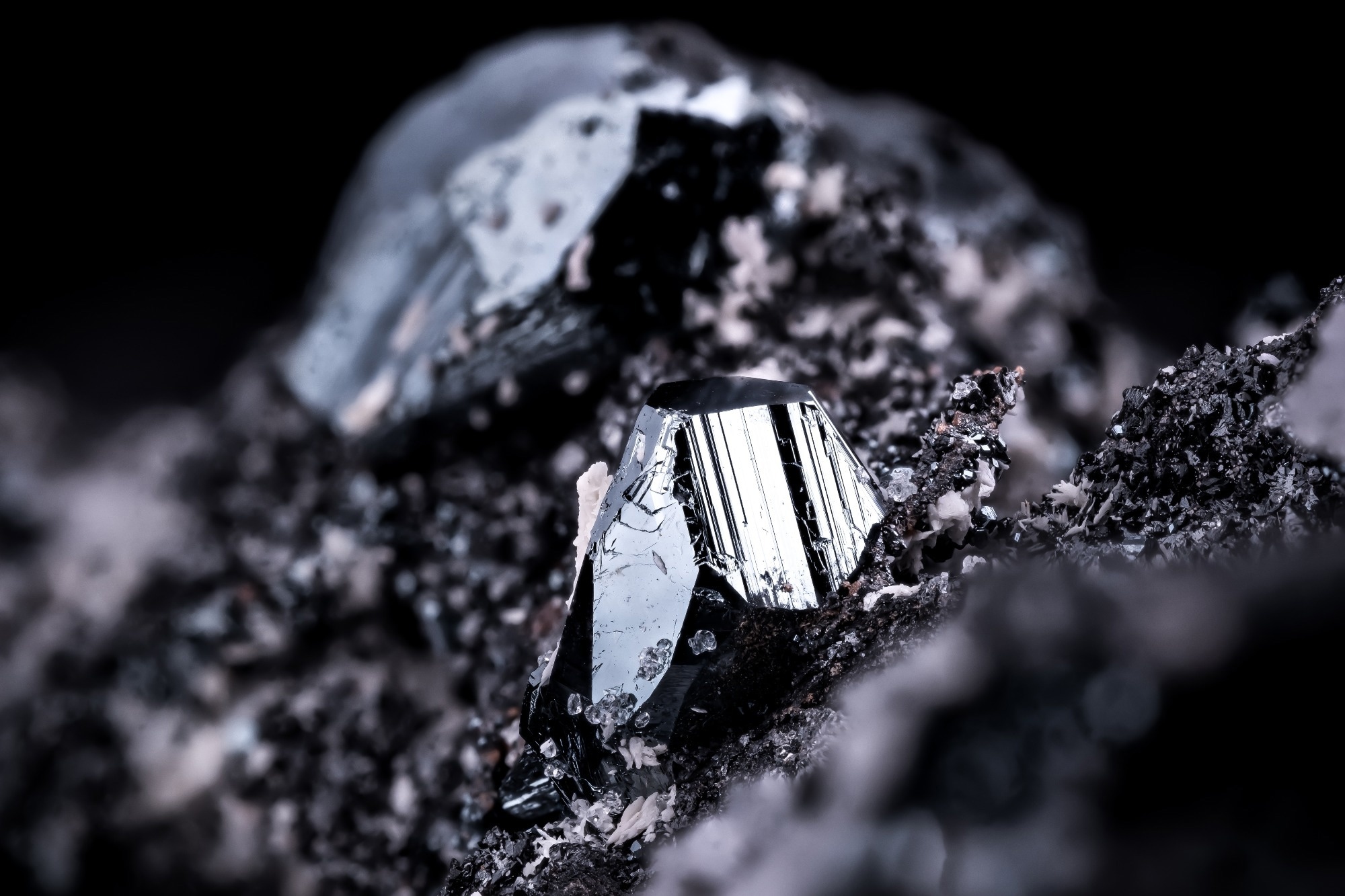
Image Credit: mineral vision/Shutterstock.com
Hematite’s Magnetic Properties
The magnetic properties of hematite are characterized by three distinct temperatures. These are the Néel temperature, the Morin temperature, and the blocking temperature.
Studies have revealed the Néel temperature of bulk hematite to be around 960 K. Below its Néel temperature, hematite behaves as an antiferromagnetic material, with magnetic moments aligning in opposite directions. This alignment cancels out the net magnetic effect, resulting in a total magnetic moment of zero.
The Morin transition temperature has been estimated to be around 263K, with the magnetic spin being antiparallel below the Morin temperature, leading to the uniaxial antiferromagnetic properties displayed by the hematite. As the temperature exceeds the Morin temperature, a slight magnetic moment is recorded, leading to weak ferromagnetism. If the size of the particles decreases, a decrease is observed in the Morin temperature.
After a certain limit, a further reduction in particle size leads to a fluctuation in the reorientation of the magnetic moment in a single domain. This is due to the thermal agitation, which leads to the hematite behaving like a superparamagnetic material.3
No time to read now? Download your PDF copy!
Spintronics and Sustainable Computing
Spintronics plays a central role in the development of next-generation computational platforms and nanoelectronic devices. It offers a promising approach to optimizing energy efficiency while significantly enhancing storage capacity and computational speed. These devices utilize the spin degrees of freedom of electrons, where the spin polarization can be regulated by magnetic layers or spin-orbit coupling.4
Rare and toxic elements, as well as molecular semiconductors (MSCs), have been used traditionally. However, the effect of physicochemical structures of MSCs on spin relaxation and magnetic properties is very unclear, leading to major challenges in selecting highly efficient materials for spintronics applications.
Furthermore, the conventional materials exhibit shorter lifespan and unfavorable spin-transport efficiency, making it necessary to look for alternatives.5 In this regard, hematite has emerged as a sustainable and highly abundant material with much higher power efficiency, becoming popular among the experts researching spintronic devices.
Room-Temperature Operation Advantage
The net-zero magnetization and resistance to external magnetic fields make antiferromagnets especially valuable for ultra-fast, energy-efficient spintronic devices. These properties are critical for developing advanced frameworks for next-generation information processing and storage systems. For everyday utilization, efficient operations at room temperature become a necessity.6
Hematite, characterized by a corundum crystalline structure, is antiferromagnetic below the high Néel temperature of 675℃. Around room temperature, the spin moments are perpendicular to the basal plane, making it a true antiferromagnet.7 This makes hematite a highly promising candidate for practical implementation in computing platforms.
The control of spin currents is at the base of spintronics, and the spin current in antiferromagnets can be characterized as having opposite polarization. The ability to control spin currents in hematite at room temperature—without the need for extensive cooling—has already been demonstrated.8 This capability is helping to open up new possibilities in the emerging field of antiferromagnetic spintronics..
Integration with Existing Technologies
Hematite is easily fabricated by conventional ore processing techniques like hydrothermal synthesis and electrochemical methods. No specialized equipment is required for processing hematite, making the process economical. The current semiconductor manufacturing techniques can be easily used to fabricate hematite-based semiconductor devices used in logic gates and neuromorphic computing platforms. Chemical precipitation techniques have been successfully demonstrated to produce hematite-based nanoparticles, including metal-doped nanoparticles.9 The compatibility with established manufacturing methods makes hematite an economical choice.
Environmental and Ecological Benefits
Rare earth metals are essential for microelectronics and computing devices. These metals are available in very scarce concentrations, and an individual ore doesn’t contain a single rare metal; rather, a complex mixture is present, making specialized separation techniques a requirement.
Rare metals are associated with radioactive substances, making their mining a hazardous process leading to exceptional waste generation, and releasing pollutants responsible for water, soil, and air pollution. These metals severely affect the water quality, disturbing the natural ecosystem as well as causing irreparable damage to the environment and the living organisms 10
In comparison, hematite is a purely iron oxide ore, not associated with any radioactive pollutants. It is abundantly present and is easily processed using conventional techniques, making it highly economical. Experts have also demonstrated a sustainable, eco-friendly, and highly efficient fabrication method of producing hematite nanoparticles in many studies using organic raw materials.11 Hematite’s potential for reuse and recycling offers a promising path toward accelerating sustainable electronics manufacturing and advancing the carbon-neutral production of semiconducting devices.
Challenges and Research Direction
Experts have recently discovered that hematite photo-electronic devices have a significant limitation and are unable to develop a sufficient Fermi level splitting under light. This is because of the reduction of Fe from Fe3+ to Fe2+ due to the formation of electron polarons. This is a major issue and will need to be resolved efficiently and economically.12
There is much ongoing research focused on developing some modified and/or novel approaches for meticulously controlling the nanostructure and composition of hematite, as these two main factors substantially affect the electronic structure and physicochemical performance of microelectronics.13 In this regard, interfacial engineering is a popular approach, and the implementation of Machine learning algorithms is expected to boost the process of developing highly optimized functional hematite materials as well as understanding spin injection mechanisms in hematite material.
These key steps will make sure that hematite is at the forefront of the development of next-generation fast and efficient computing frameworks.
Further Reading
- Eggleston, C. (2008). Toward new uses for hematite. Science. 320(5873), 184-185. Available at: https://doi.org/10.1126/science.1157189
- University of Minnesota. (2025). Hematite: Oxides mineral group. [Online]. Available at: https://commonminerals.esci.umn.edu/minerals-g-m/hematite [Accessed on: May 05, 2025].
- Tadić, M. et. al. (2011). Synthesis, morphology, microstructure and magnetic properties of hematite submicron particles. Journal of alloys and compounds, 509(28), 7639-7644. Available at: https://doi.org/10.1016/j.jallcom.2011.04.117
- Hirohata, A. et. al. (2020). Review on spintronics: Principles and device applications. Journal of Magnetism and Magnetic Materials, 509, 166711. Available at: https://doi.org/10.1016/j.jmmm.2020.166711
- Gu, X. et. al. (2023). Challenges and prospects of molecular spintronics. Precision chemistry. 2(1). 1-13. Available at: https://doi.org/10.1021/prechem.3c00071
- U.S. National Science Foundation. (2025). Antiferromagnetic hybrids achieve important functionality for spintronic applications. [Online]. Available at: https://www.nsf.gov/news/antiferromagnetic-hybrids-achieve-important [Accessed on: May 06, 2025].
- Jiang, Z. et. al. (2014). Ferro and antiferromagnetism of ultrafine‐grained hematite. Geochemistry, Geophysics, Geosystems, 15(6), 2699-2712. Available at: https://doi.org/10.1002/2014GC005377
- Sheng, L. et. al. (2025). Controlling spin currents with magnon interference in a canted antiferromagnet. arXiv preprint arXiv:2502.17769. Available at: https://doi.org/10.48550/arXiv.2502.17769
- Rajapandi, P. et. al. (2023). Influence of Ni doping on hematite nanoparticles for enhanced structural, optical, magnetic properties and antibacterial analysis. Journal of Molecular Structure. 1284. 135397. Available at: https://doi.org/10.1016/j.molstruc.2023.135397
- Feffer, J. (2023). Mapping the Impact and Conflicts of Rare-Earth Elements. Institute for Policy Studies. [Online]. Available at: https://ips-dc.org/mapping-the-impact-and-conflicts-of-rare-earth-elements/ [Accessed on: May 07, 2025].
- Zhu, S. et. al. (2020). Green sustainable and highly efficient hematite nanoparticles modified biochar-clay granular composite for Cr (VI) removal and related mechanism. Journal of Cleaner Production. 276. 123009. Available at: https://doi.org/10.1016/j.jclepro.2020.123009
- Lohaus, C. et. al. (2018). Limitation of Fermi level shifts by polaron defect states in hematite photoelectrodes. Nat Commun 9, 4309. Available at: https://doi.org/10.1038/s41467-018-06838-2
- Wan, H. et. al. (2023). Advanced hematite nanomaterials for newly emerging applications. Chemical Science, 14(11), 2776-2798. Available at: https://doi.org/10.1039/D3SC00180F
Disclaimer: The views expressed here are those of the author expressed in their private capacity and do not necessarily represent the views of AZoM.com Limited T/A AZoNetwork the owner and operator of this website. This disclaimer forms part of the Terms and conditions of use of this website.