By Ankit SinghReviewed by Susha Cheriyedath, M.Sc.Apr 11 2024
In today's digital age, secure communication is essential, with cryptography serving as the cornerstone of safeguarding sensitive information. Traditional cryptographic methods rely on complex mathematical algorithms for protection. However, the rise of quantum computers threatens their effectiveness. Fortunately, quantum encryption, also known as quantum cryptography, presents a groundbreaking solution to this challenge.
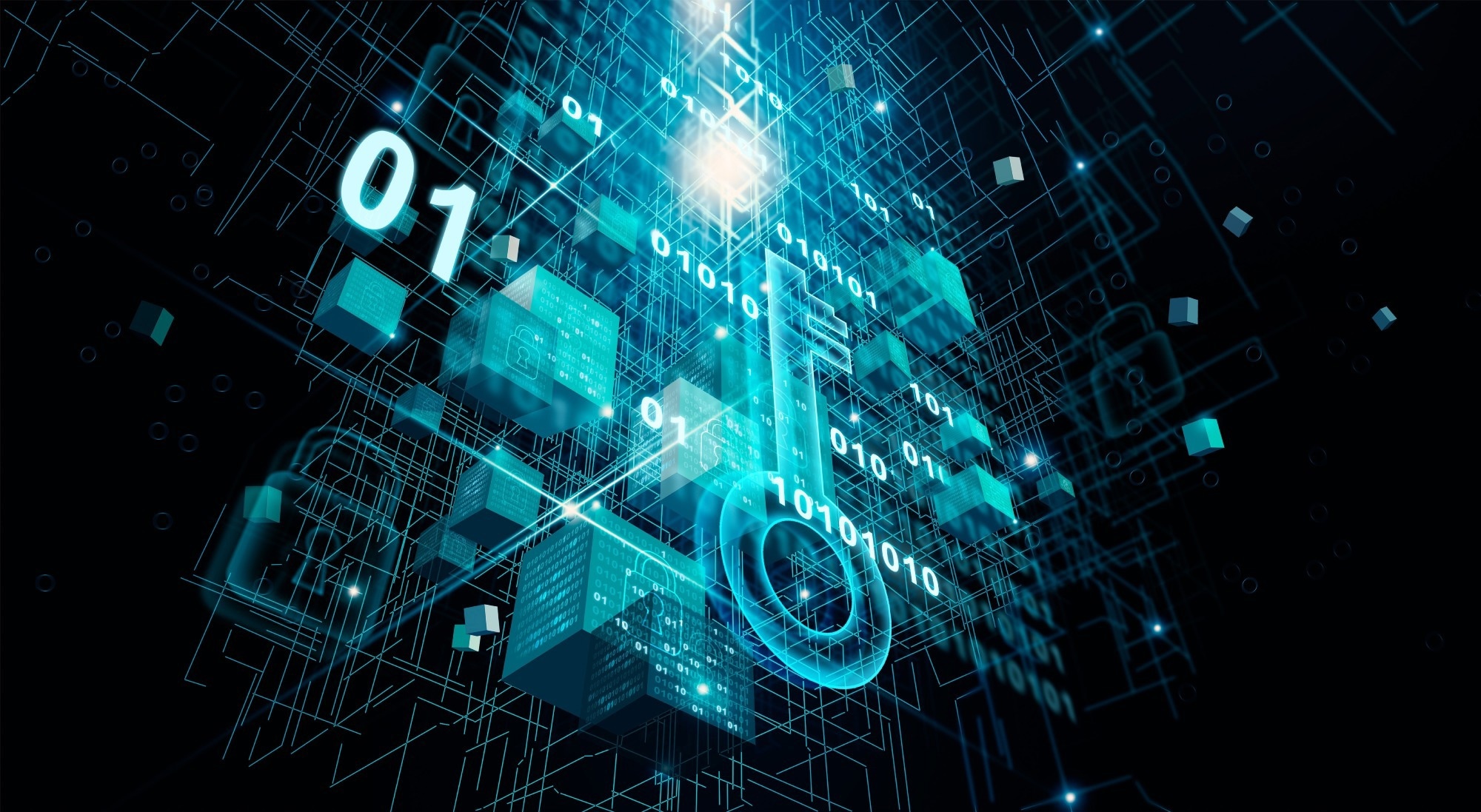
Image Credit: PopTika/Shutterstock.com
Quantum encryption makes use of the laws of quantum mechanics to create unbreakable communication channels. It exploits phenomena like superposition (where particles exist in multiple states simultaneously) and entanglement (where linked particles share a common fate) to identify any attempts at eavesdropping.1,2
This article delves into the latest developments in the field of quantum encryption and its potential applications across domains. It also discusses concerns related to the implementation and scalability of this technology, underscoring the need for further research and development to fully unlock its capabilities.
Evolution of Quantum Encryption
The idea of quantum encryption dates back to the 1970s when Stephen Wiesner developed innovative ideas for quantum coding and quantum money. However, the fundamental groundwork for quantum encryption was laid in 1984 by Charles Bennett and Gilles Brassard. They introduced the concept of quantum key distribution (QKD), which centered on creating a secure encryption key by leveraging the unpredictable behavior of quantum particles rather than directly encrypting the data. The initial protocols for QKD relied on photons to detect eavesdropping by exploiting properties such as superposition and entanglement.1
Researchers like Artur Ekert have introduced new protocols based on Bell's inequalities, strengthening the field's theoretical foundations. Initially, the focus was on overcoming technical challenges, as early QKD systems were large and costly. However, improvements in photonics and the miniaturization of quantum devices have led to increased efficiency and scalability. 2
researchers are achieving unprecedented secure key rates and transmission distances while also exploring alternative platforms such as entangled microwave photons for long-distance communication. This ongoing evolution, fueled by tireless research endeavors, is laying the groundwork for a future where quantum encryption becomes a practical reality.
Applications of Quantum Encryption
Quantum encryption presents significant potential in diverse domains, especially in ensuring secure communication networks. Leveraging the distinct properties of quantum mechanics like superposition and entanglement, it guarantees the confidentiality and integrity of transmitted data. This technology finds applications in sectors such as finance, healthcare, government, and defense, where safeguarding sensitive information is crucial.
In the finance sector, banks and financial institutions handle highly sensitive data. Quantum encryption offers a robust solution to protect online transactions, interbank communication, and customer information, shielding financial data from unauthorized access and manipulation.2,4
Quantum encryption plays a crucial role in safeguarding infrastructure systems such as power grids, transportation networks, and communication systems against cyberattacks, thereby preventing disruptions.2 Additionally, government agencies and defense organizations utilize quantum encryption to secure classified communications and critical infrastructure from cyber threats and espionage.2
Healthcare organizations can leverage quantum encryption to safeguard electronic medical records and patient information, ensuring both privacy and compliance with data protection regulations. A recent study published in the Journal of Medical Internet Research explores the potential of quantum cryptography for protecting electronic health information, highlighting its role in protecting patient privacy and ensuring trust in healthcare systems.9
As quantum technology advances, the applications of quantum cryptography are expected to expand to more complex areas, for example, to create secure voting systems, protect intellectual property, and enable secure communication for autonomous vehicles.
Advantages of Quantum Encryption
Quantum encryption offers several advantages over traditional cryptographic methods. Chiefly, it provides unbreakable security, unlike conventional techniques that depend on the complexity of factoring large prime numbers—a vulnerability quantum computers could exploit. In contrast, quantum encryption relies on the fundamental laws of physics, theoretically making it impervious to decryption attempts.4,7
Secondly, quantum encryption provides tamper detection. Any attempt to intercept or tamper with quantum communication will disrupt the entangled particles, alerting the sender and receiver. This provides an additional layer of security compared to traditional methods.2,4
Moreover, quantum encryption facilitates the secure distribution of keys over extensive distances via quantum entanglement, thereby establishing the groundwork for global-scale secure communication networks. This functionality holds particular value in scenarios where conventional key exchange mechanisms are vulnerable to interception or tampering.4
Challenges and Concerns
Although promising, quantum encryption encounters several challenges hindering its widespread adoption. One notable obstacle is scalability and compatibility with current infrastructure. Integrating quantum encryption protocols necessitates specialized hardware and infrastructure, potentially posing logistical and financial hurdles for organizations transitioning from classical to quantum-secure systems. Furthermore, quantum communication is optimized for fiber optic cables, constraining its reach and posing challenges for sending quantum signals over extended distances.2,5
Additionally, quantum encryption methods are susceptible to implementation flaws and technical limitations. Practical implementations often face challenges such as quantum noise, channel loss, and photon detection efficiency, which can undermine the security and reliability of quantum-secure communication systems.2
Researchers are actively addressing these challenges, and they believe that advancements in photonics, miniaturization of quantum devices, and the development of new protocols will pave the way for a more practical and scalable future for quantum encryption.
Latest Research and Developments
Recent progress in quantum encryption research has been aimed at overcoming current obstacles and extending the practical uses of quantum-secured communication. Significant breakthroughs include the investigation of innovative QKD protocols that provide improved security and efficiency.2,3
In a recent study, researchers demonstrated a new QKD protocol that achieved a record-breaking secure key rate of up to 50 kilometers over standard telecom fibers.2 Another study published in Nature Photonics demonstrated a new QKD protocol that exceeded secure key rates of 110 Mbps over a distance of 10 kilometers. This represents a key milestone in quantum encryption and brings practical deployments in real-world scenarios closer to reality.8
In another significant advancement, researchers from the University of Science and Technology of China achieved a groundbreaking milestone in secure key distribution using a satellite-based quantum communication link. The team successfully distributed secure keys over an unprecedented distance of 1200 kilometers at a rate of 0.0034 bits per second. This achievement showcases the potential for establishing secure quantum communication infrastructure over extensive distances.6
Researchers at the Los Alamos National Laboratory are developing a quantum network based on entangled microwave photons. This network has the potential to enable long-distance quantum communication, opening up new possibilities for secure communication and data transmission.2
Simultaneously, the National Institute of Standards and Technology (NIST) is diligently working to standardize cryptographic algorithms resistant to quantum computing. This effort aims to streamline the transition to post-quantum cryptography in the future, ensuring robust security measures.
Furthermore, recent strides in quantum error correction techniques have bolstered the resilience of quantum encryption against noise and imperfections in real-world quantum systems.10 These advancements indicate that quantum encryption is transitioning from theoretical promise to practical implementation.
Researchers are actively addressing technical challenges, including the reduction of complexity and cost associated with quantum devices, extension of the reach of quantum communication networks, and seamless integration with existing classical communication infrastructure. Such endeavors underscore ongoing efforts to advance the feasibility and efficacy of quantum encryption in practical applications.
Future Prospects and Conclusion
The future of quantum encryption holds immense promise as researchers continue to push the boundaries of quantum technology. With ongoing efforts to enhance the scalability, reliability, and practicality of quantum encryption protocols, the prospect of a quantum-secure internet ecosystem is becoming increasingly attainable.2,4
Furthermore, the emergence of quantum computing has presented both a challenge and an opportunity for quantum encryption. While traditional cryptographic systems are vulnerable to quantum computers, the development of new cryptographic primitives and protocols that are resilient to quantum attacks is now possible. As quantum encryption becomes more accessible, it is poised to revolutionize cybersecurity across industries, ushering in a new era of secure and trustworthy communication.
In conclusion, quantum encryption is a cutting-edge cybersecurity technology that provides unmatched security and protection against the evolving threats posed by quantum computing. Despite obstacles, constant research and innovation are vital in unlocking the full capabilities of quantum encryption. This will bring in a future where the principles of quantum mechanics will ensure the privacy and security of data.
References and Further Reading
- Bennett, C. H., & Brassard, G. (1984). Quantum cryptography: Public key distribution and coin tossing. Proceedings of IEEE International Conference on Computers, Systems and Signal Processing, 175-179. https://doi.org/10.48550/arXiv.2003.06557
- Vatsal Vasani, Kumar Prateek, Ruhul Amin, Soumyadev Maity, Ashutosh Dhar Dwivedi, Embracing the quantum frontier: Investigating quantum communication, cryptography, applications and future directions, Journal of Industrial Information Integration, 39, 2024, 100594. https://doi.org/10.1016/j.jii.2024.100594
- Yin, J., Cao, Y., Li, Y. H., Liao, S. K., Zhang, L., Ren, J. G., & Pan, J. W. (2022). Quantum communication with synchronous satellites. Nature, 596(7871), 548-553.
- Pirandola, S., & Andersen, U. L. (2020). Quantum cryptography. Reviews of Modern Physics, 92(3), 035002.
- Lucamarini, M., & Yuan, Z. L. (2021). Practical challenges in quantum key distribution. Light: Science & Applications, 10(1), 1-15. https://doi.org/10.48550/arXiv.1606.05853
- Liao, S. K., Li, Y. H., Yin, J., Zhao, Q., Liu, H., Zhang, L., & Pan, J. W. (2017). Satellite-to-ground quantum key distribution. Nature, 549(7670), 43-47. https://doi.org/10.1038/nature23655
- Pirandola, S., & Weedbrook, C. (2019). Advantages of coherent protocols for Gaussian quantum key distribution. Physical Review X, 9(3), 031065.
- Li, W., Zhang, L., Tan, H. et al. High-rate quantum key distribution exceeding 110 Mb s–1. Nat. Photon. 17, 416–421 (2023). https://doi.org/10.48550/arXiv.2307.02364
- Chen Y, Esmaeilzadeh P. Generative AI in Medical Practice: In-Depth Exploration of Privacy and Security Challenges J Med Internet Res 2024;26:e53008. https://doi.org/10.2196/53008
- NIST to Standardize Encryption Algorithms That Can Resist Attack by Quantum Computers. (2023, August 24). NIST.
Disclaimer: The views expressed here are those of the author expressed in their private capacity and do not necessarily represent the views of AZoM.com Limited T/A AZoNetwork the owner and operator of this website. This disclaimer forms part of the Terms and conditions of use of this website.