By Samudrapom DamReviewed by Susha Cheriyedath, M.Sc.Updated on Nov 5 2024
Quantum chemistry is the application of quantum mechanics to understand the subtleties of chemical and physical behavior at the atomic and molecular level. It is a field that allows us to explore the structure and dynamics of molecules, the flow of charge and energy, and the interactions of matter with light—shedding light on the mechanisms that drive everything from chemical bonding to reaction kinetics.1,2
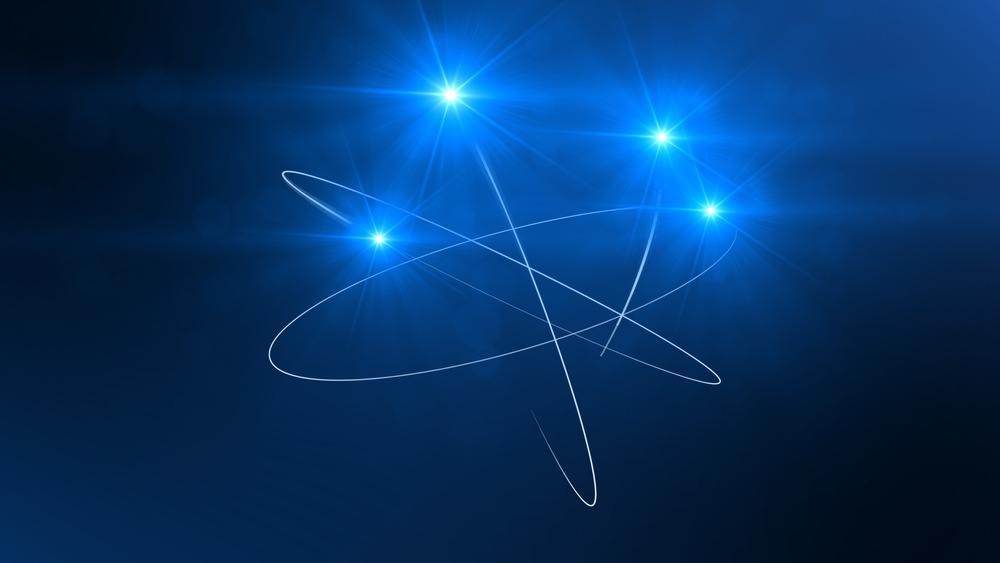
Image Credit: alexnako/Shutterstock.com
Quantum chemistry spans a wide range of phenomena, from the electronic structure of matter and its interactions with light to the flow of charge and energy within molecular systems. It explores the collective behaviors of complex ensembles and the dynamic quantum processes of evolving systems.
To study these quantum properties at the atomic and molecular levels, quantum chemists employ a combination of chemical synthesis, spectroscopy, and theoretical modeling. Through these approaches, they are able to gain a deeper understanding of the underlying quantum mechanics that drive chemical and physical transformations..1,2
The Basics of Quantum Mechanics
In quantum chemistry, key quantum mechanics concepts like quantum states, the uncertainty principle, and wave-particle duality provide insights into the electronic structure and properties of atoms and molecules.3-5
The Heisenberg uncertainty principle limits the accuracy with which a particle's position and momentum are known simultaneously. This principle applies to various pairs of variables, but the most common involves position and momentum. Quantum-mechanical operators are used to rigorously prove this principle.3,4
Quantum mechanics also describes how extremely small objects simultaneously exhibit the characteristics of waves (a variation or disturbance that transfers energy) and particles (tiny pieces of matter). This is known as wave-particle duality.3,4
A sub-microscopic system, like a sub-atomic particle/set of sub-atomic particles exerting a force on each other/moving under a force field, is described by a quantum state. The state is a list of the system’s physical properties that are measured simultaneously. Yet, the particle momentum or position could specify the state for a free point particle, but not both simultaneously, as dictated by Heisenberg's uncertainty principle.4,5
The Fundamentals of Quantum Science
Quantum Chemistry vs Classical Chemistry
Quantum chemistry and classical chemistry represent two distinct approaches to understanding the behavior of matter. Classical chemistry is rooted in Newtonian mechanics, the most popular classical mechanics formalism, and describes the macroscopic world, treating atoms and molecules as discrete particles with well-defined properties.6,7
Classical mechanics assumes that properties like energy, momentum, and position can vary continuously. Additionally, system behavior can be precisely predicted based on initial conditions.6,7
Quantum chemistry, on the other hand, focuses more on the microscopic realm, where quantum mechanics principles govern the behavior of subatomic particles. It offers a more accurate description of chemical phenomena, specifically those involving the electronic structure of atoms and molecules.6,7
Quantum chemistry recognizes that specific properties like energy are quantized, implying they only exist in discrete values. Additionally, it utilizes probability to describe the behavior of particles/ Heisenberg's uncertainty principle.6,7
Key Concepts in Quantum Chemistry
Understanding the quantum nature of matter starts with a few fundamental ideas that quantum chemists work with regularly—atomic orbitals, electron clouds, and probability distributions. These core concepts are the language of quantum chemistry, helping us to model and understand matter at its most fundamental level.8-10
Atomic Orbitals
Unlike the classical notion of electrons orbiting the nucleus in defined paths, quantum mechanics describes electrons as existing within orbitals, which are probability regions rather than fixed trajectories. Each orbital type—s, p, d, and f—arises from different angular momentum quantum numbers, labeled 0 through 3, respectively.
An s-orbital, for instance, has a spherical probability distribution, while p-orbitals take on a dumbbell shape, and d-orbitals have even more complex structures. These shapes represent where you’re likely to find an electron around the nucleus.
Visualizing orbitals typically involves boundary surfaces that mark regions with about a 90 % probability of containing the electron. This is not about exactness—quantum mechanics does not allow for pinpointing an electron’s exact location. Instead, it is about capturing the regions where the electron “spends” most of its time. You might see signs like + and - attached to different parts of an orbital to indicate the wavefunction’s phase, but in practice, the precise shape of the probability density is often simplified for clarity.8
Electron Cloud
Rather than thinking of electrons as discrete points, it is often more accurate to picture them as forming an “electron cloud” around the nucleus. This cloud has varying density—higher density indicates a higher probability of finding the electron. The idea of an orbital helps us map out this cloud in 3D space, giving us a sense of where an electron is most likely to be at any given time. Quantum mechanics shows us that electrons are not in fixed orbits; they occupy regions where they are more likely to be, with denser parts of the cloud indicating higher probability zones.9
Probability Distributions
Due to De Broglie’s wave-particle duality, electrons and other particles do not travel in exact paths but instead show probabilistic patterns of location. When we measure a particle repeatedly, we start to see a distribution emerge based on its wave-like nature, which reflects where the particle is statistically likely to be found. This probability distribution forms the basis for understanding electron configurations in atoms and molecules. It’s not that the particle’s path is random but that quantum mechanics only allows us to describe likely positions rather than specific ones.10
The Role of Schrödinger's Equation in Quantum Chemistry
The Schrödinger equation is a mathematical model for particles like electrons that simultaneously behave as waves. It is a fundamental equation in quantum mechanics that describes the electron behavior within atoms and molecules. It is a differential equation that provides information about the probability of finding a particle in a specific location.11,12
The solutions to this equation, often visualized as three-dimensional graphs, are known as orbitals/electron clouds. Schrödinger equation is the key to understanding chemical bonding, reaction mechanisms, and material properties at the quantum level, bridging quantum mechanics with chemical theory.11,12
One of the most significant applications of the Schrödinger equation is in understanding the hydrogen atom. This simple system can be solved analytically, providing valuable insights into atomic structure and quantum mechanics as a whole.11,12
Applications of Quantum Chemistry in Modern Science
In modern science, major applications of quantum chemistry in nanotechnology, drug design, and materials science include:13
- The study of larger systems with more approximate methods
- The simulation of polymers and surfaces and interactions of surfaces
- Modeling the interaction between possible drugs and enzyme-active sites
- The prediction of structures and spectra of possible copper (I) probes
- Designing of new molecules
- Understanding molecules that are hard to study experimentally/highly reactive molecules
- Understanding electronic properties and identifying potential energy surfaces
A Quantum Future: How Quantum Applications are Disrupting Key Sectors
Challenges and Future Directions in Quantum Chemistry
Quantum chemistry has made remarkable advances in bridging quantum mechanics and chemistry, but key challenges remain. Modeling molecular states with high accuracy is particularly difficult, especially for complex or large systems. Quantum effects become exponentially harder to compute as the number of interacting particles grows, which can limit our ability to model and predict molecular behaviors precisely.14,15
Despite these hurdles, the field still has huge potential. Emerging technologies, especially artificial intelligence (AI) and machine learning (ML) are opening new doors. AI can help process vast amounts of data and identify patterns that may be too complex for traditional methods, enabling us to approach problems like material design, drug discovery, and energy storage with a new level of precision.
As computational power and algorithms continue to improve, quantum chemistry is set to play an increasingly central role in shaping the future of science and technology.
Building the Future with Quantum Materials
References and Further Reading
- Quantum Chemistry [Online] Available at https://pme.uchicago.edu/quantum-uchicago-research/quantum-chemistry (Accessed on 04 November 2024)
- Lashkaripour, A. (2021). Introduction to Quantum Chemistry: The Schrödinger Equation. https://www.researchgate.net/publication/350175449_Introduction_to_Quantum_Chemistry_The_Schrodinger_Equation
- Introduction to quantum mechanics [Online] Available at https://scholar.harvard.edu/files/david-morin/files/waves_quantum.pdf (Accessed on 04 November 2024)
- DOE Explains...Quantum Mechanics [Online] Available at https://www.energy.gov/science/doe-explainsquantum-mechanics (Accessed on 04 November 2024)
- Quantum States and Superposition [Online] Available at https://www.southampton.ac.uk/~doug/quantum_physics/superposition.pdf (Accessed on 04 November 2024)
- Quantum vs. Classical [Online] Available at https://quantumatlas.umd.edu/entry/quantum-classical/ (Accessed on 04 November 2024)
- Physics Classifications: Classical and Quantum Mechanics [Online] Available at https://alan.ece.gatech.edu/ECE6451/Lectures/ECE6451L2ClassicalQuantumMechanicsReviewAndLimitations.pdf (Accessed on 04 November 2024)
- Molecular Quantum Mechanics [Online] Available at https://web.stanford.edu/~oas/SI/QM/Atkins05.pdf (Accessed on 04 November 2024)
- Quantum Mechanical Atomic Model [Online] Available at https://chem.libretexts.org/Bookshelves/Introductory_Chemistry/Introductory_Chemistry_(CK-12)/05%3A_Electrons_in_Atoms/5.11%3A_Quantum_Mechanical_Atomic_Model (Accessed on 04 November 2024)
- The Heisenberg Uncertainty Principle [Online] Available at https://chem.libretexts.org/Courses/BethuneCookman_University/B-CU%3ACH-331_Physical_Chemistry_I/CH-331_Text/CH-331_Text/01%3A_The_Dawn_of_the_Quantum_Theory/1.9%3A_The_Heisenberg_Uncertainty_Principle (Accessed on 04 November 2024)
- Karnik, A. V., & Hasan, M. (2021). Pericyclic reactions. Stereochemistry, 515-550. DOI: 10.1016/B978-0-12-821062-8.00006-5, https://www.sciencedirect.com/science/article/abs/pii/B9780128210628000065
- The Schrödinger Equation [Online] Available at https://math.libretexts.org/Bookshelves/Differential_Equations/Differential_Equations_(Chasnov)/09%3A_Partial_Differential_Equations/9.08%3A_The_Schrodinger_Equation (Accessed on 04 November 2024)
- Applications of Quantum Chemistry [Online] Available at http://vergil.chemistry.gatech.edu/courses/chem3412/handouts/Applications_of_Quantum_Chemistry.pdf (Accessed on 04 November 2024)
- Ito, Y. et al. (2024). Introduction to Computational Quantum Chemistry for Computer Scientists. 2024 IEEE International Parallel and Distributed Processing Symposium Workshops (IPDPSW), 273-282. DOI: 10.1109/IPDPSW63119.2024.00066, https://ieeexplore.ieee.org/abstract/document/10596489
- AI tackles one of the most difficult challenges in quantum chemistry [Online] Available at https://www.imperial.ac.uk/news/255673/ai-tackles-most-difficult-challenges-quantum/ (Accessed on 04 November 2024)
Disclaimer: The views expressed here are those of the author expressed in their private capacity and do not necessarily represent the views of AZoM.com Limited T/A AZoNetwork the owner and operator of this website. This disclaimer forms part of the Terms and conditions of use of this website.