AZoQuantum talks to Dr. Stefano Bagnulo about his research relating to the magnetism of white dwarfs.
Could you please tell us about your background in astronomical science and how you came to be involved with this research?
I have been interested in the magnetic fields of stars since I was a student at university in Florence, originally relating to the theoretical side. I then acquired expertise in observations of magnetism when I was a staff astronomer at the Very Large Telescope (VLT) of the European Southern Observatory (ESO). One of the instruments of the ESO VLT, called FORS, was specifically designed in part to make possible the study of magnetic white dwarfs, and it seemed natural for me to start to use this instrument for the measurements of stellar magnetic fields in general, and of white dwarfs in particular.
Can you please explain what a white dwarf is? What stage in the lifecycle of the star are they, and why do they form?
The white dwarf stage is the final stage of the life of more than 90% of stars. It is not easy to summarize stellar evolution in a few sentences, but the main point is that in a star there are two main forces acting: the material that composes a star is kept together by the gravitational force, which tends to shrink the star. In the interior of the star, the gas is very hot, producing pressure that counteracts gravity and supports the outer layers of the star.
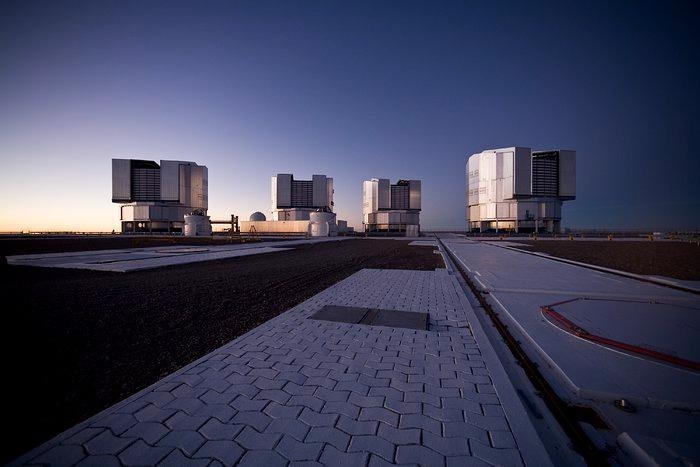
The four Unit Telescopes at the European Southern Observatory. Image Credit: ESO/H.H.Heyer
However, this heat energy leaks out as the star shines. The lost energy is replaced for a long time by nuclear reactions which replace the lost heat and prevent the pressure from dropping. However, when the fuel is exhausted, the star eventually collapses to become a kind of very high density solid or fluid that supports itself against gravity by being strong rather than hot. This requires the star to be much smaller and denser than it was before.
At the end of its life, a star like our Sun, for instance, will become the size of Earth (reducing its radius by a factor of 100!). In term of density, imagine that the mass of a big car would be compressed to the volume of a tablespoon. Although energy is no longer produced, a white dwarf will continue to emit radiation (and be visible) because it is still very hot inside, and this heat slowly leaks out and is radiated into space; with time the white dwarf will cool down until it will become practically invisible.
This cooling process may take more than 10 billion years.
Younger white dwarfs have been described to be easier to observe. Could you explain why?
A young white dwarf is still very hot inside, around 10 million degrees. This heat leaks out, making the white dwarf visible. As the interior of the white dwarf cools, heat leaks out more slowly and the star gradually becomes fainter as it ages, like a cooling piece of iron removed from the blacksmith’s fire.
What techniques are used to observe and analyze stellar magnetic fields?
The presence of a magnetic field in a stellar atmosphere affects the way in which the light travels through the stellar atmosphere until it is radiated out into space. We say that the light is polarized. Polarization can be measured with special instruments called “polarimeters”, which are complicated versions of polarizing sunglasses. The presence of a certain kind of polarization is a sure sign of a magnetic field. We can also look at “spectral lines” in the light from white dwarfs.
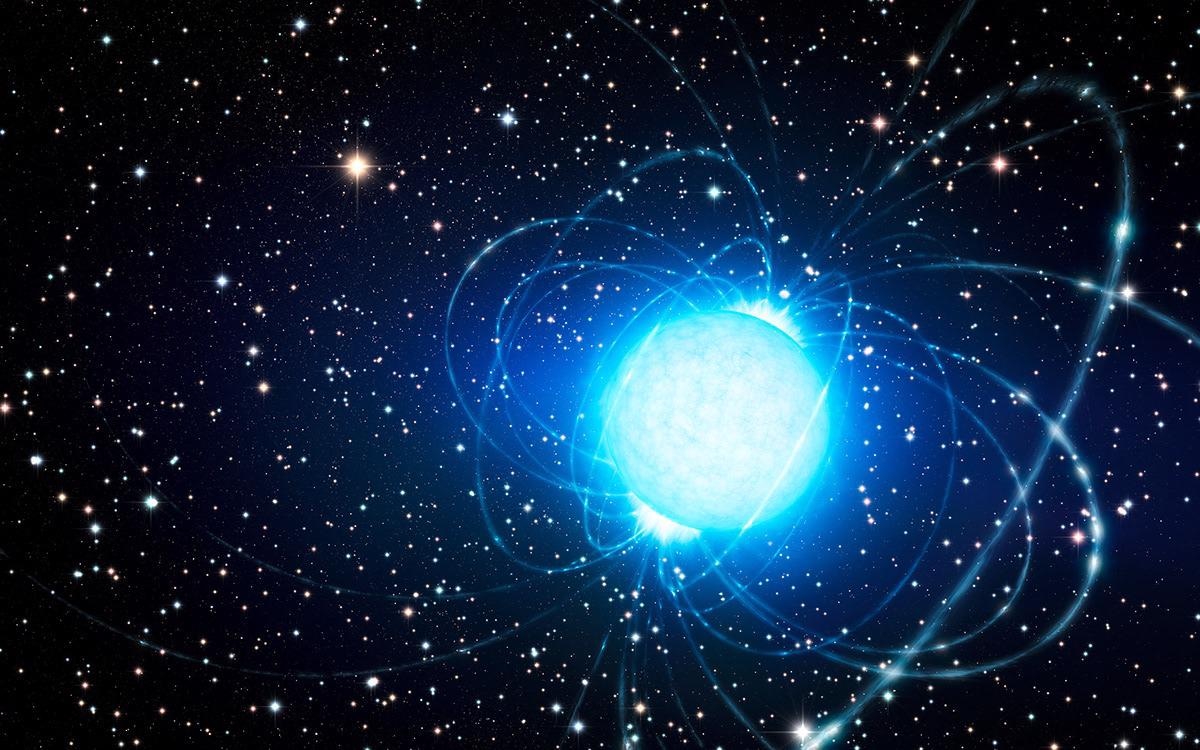
Artist rendering of a Magnetar. Image Credit: ESO/L. Calçada
These are particular single colors that are greatly weakened in the starlight because they are absorbed by atoms such as those of hydrogen. When a strong magnetic field is present, a symptom is that the single color absorbed splits into three slightly different colors that are absorbed. This effect can sometimes be detected by the ordinary spectroscopy widely used to study white dwarfs.
What were the parameters of your research and experiment, and how was it conducted?
We wanted to look for correlations between the occurrence of magnetic fields and other stellar parameters, in particular the age. We wanted to check, for example, whether magnetic fields get weaker with time, as we found for another category of magnetic stars, the Ap and Bp stars. We soon realized that the data available in the literature were strongly biased in favor of the youngest stars with the strongest fields, and could not provide a good overview of the general situation.
What we needed was a sample that was representative of the entire population of white dwarfs. Naively, one could think to go to a telescope and observe as many stars as possible, starting from the brightest ones (as they are easier to observe), probably leaving out the faintest ones.
But from what we said earlier, the brightest stars are often also the youngest ones, so that older stars would be vastly underrepresented. We decided that the best experiment would be to check for magnetic fields in all white dwarfs within a certain distance from the Sun—practically, we considered a 20 parsec (about 65 light-years) radius.
The local 20pc volume contains about 150 white dwarfs. Because such a volume-limited sample is selected using a criterion independent of the star's intrinsic nature, we believe that it would be much more representative of the entire population of white dwarfs.
Could you please tell us what your research has revealed about the time dependence of magnetism of white dwarfs? How does the frequency of magnetic fields correlate to the age of white dwarfs?
One of the most striking results is that magnetic fields are quite rare among the youngest white dwarfs and quite common in older white dwarfs. We set this age threshold at around 500 million years. This is not a totally unexpected result; in fact, some of the scientific community had suspected a long time ago that magnetic fields might be more frequent in cooler (hence older) white dwarfs than in hotter (hence younger) white dwarfs.
Our systematic search has finally supported this hypothesis with robust statistics. We also identified a puzzling trend, that magnetic fields may actually become stronger with time, but this has to be confirmed by further observations.
Do all white dwarfs become magnetic? Could you describe the proportion of magnetic white dwarfs and the factors behind this transition?
We found that about a quarter of all white dwarfs are magnetic, but in fairness, this observational evidence cannot distinguish between a situation in which all white dwarfs are magnetic for about a quarter of their lives and a situation in which only a quarter of white dwarfs is magnetic for most of their lives, or anything in between. Our observations need to be interpreted by a theory that explains the origin of the magnetic field.
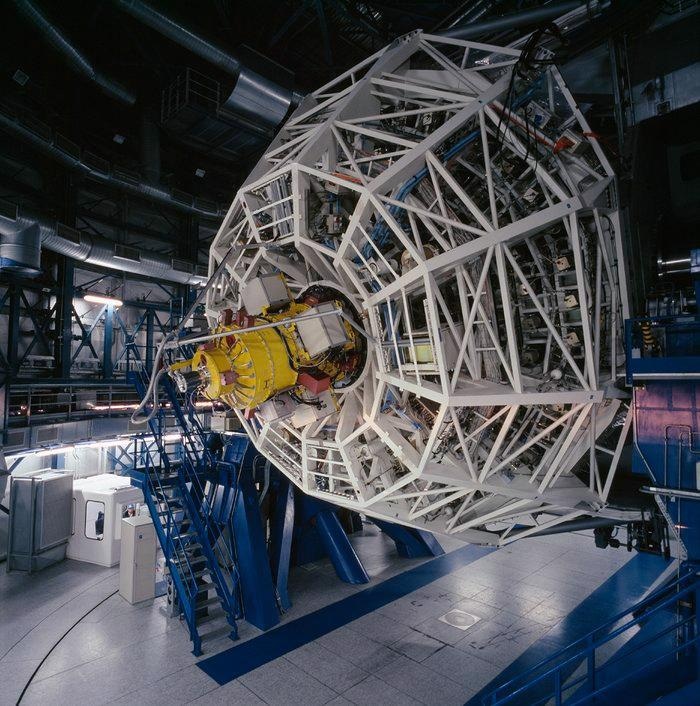
Kueyen, a second Unit Telescope, has been moved to a very low altitude, revealing the cell holding its main mirror, and the FORS1 instrument. Image Credit: ESO
Your research also confirmed something that was already well known, that is, that the average mass of the magnetic white dwarfs is higher than the average mass of the non-magnetic white dwarfs. Why is this?
One explanation concerns pairs of stars that form very close to one another, called close binary systems.
It has been suggested that two stars that formed a close binary star system may eventually merge to become a single newborn white dwarf. The rapid rotation and violent mixing in the merging process probably generate a magnetic field. The magnetic white dwarf so created has a higher mass than average because it results from the merging of two stars.
What other factors influence the occurrence and strength of magnetic fields?
The merging scenario is a plausible explanation, but we believe that there exist more mechanisms that lead to the formation of a magnetic field in a white dwarf. The problem is that we are not sure yet which ones are the most common and efficient ones; in fact, we are not even sure that we have identified all of them.
An interesting possibility is connected to a process that happens while the white dwarf is cooling down. When the star reaches a certain temperature, its core starts to solidify. As the solids sink towards the center of the star, they stir the remaining internal fluid, and if the star is spinning rapidly the result may be the generation of a magnetic field.
This mechanism is somewhat similar to the one responsible for the Earth's magnetic field. Another hypothesis that has been put forward is that a magnetic field may be generated when the debris of a planetary system falls onto the white dwarf surface, in a way similar to what would happen during the merging of two stars of a binary system.
A third possibility is that the magnetic field we see in white dwarfs is the remnant of a magnetic field that was generated in a previous stage of the life of the star. One of the first ideas that was put forward was that magnetic white dwarfs were the descendants of the magnetic Ap and Bp stars.... but this of course just shifts the question to how the magnetic field was originally formed in the white dwarf's progenitors.
What are Ap and Bp stars, and how does the magnetism of white dwarfs differ from the magnetism of these astronomical objects?
Ap and Bp stars are hydrogen burning “main sequence” stars like the Sun, but somewhat more massive. They occupy a very special role in the study of stellar magnetism, because they were the stars, apart from the Sun, in which magnetic fields were first discovered. They are the objects (other than the Sun) in which the magnetic fields are most thoroughly observed and studied.
The "p" of Ap and Bp means that these stars are "peculiar", in the sense that some chemical elements (such as iron, chromium, or rare earths) seem to be much more abundant than in the Sun. Today we understand that this peculiar surface chemistry is just apparent; the reality is that the stellar magnetic field can concentrate some chemical elements in the atmospheres where we can best see them; the highly abundant elements are no more abundant in the interiors of Ap and Bp stars than in other stars.
The magnetic fields of Ap/Bp stars share some similarities with those of white dwarfs, in that these fields do not change with time and have a smooth morphology (as opposed to the case of the solar magnetic field, which is very patchy and is concentrated in relatively small sunspots). We are not sure if they have a similar origin, though.
What are the implications of this research for understandings of the origin and evolution of a star’s magnetic field?
We have found observational constraints that cannot be explained by the current ideas—this is the classic situation in which observations guide and stimulate theorists. It will also be important to continue to systematically carry out new observations because some of the trends that we found in the local 20pc volume need to be confirmed with a larger statistical sample of stars.
What are the wider implications of this research for astrophysics and astronomical observations?
The magnetic field is a key ingredient of the recipes that describe the structure at all stellar evolutionary stages, from the time the interstellar matter collapses into a protostar to the time when the star dies, either as a white dwarf or as an exploding supernova. Up to now, magnetic fields have mostly been ignored by people working to understand how stars evolve during their lives. Our work, showing how very common these fields are in the last stages of stellar evolution, is a wake-up call to the theory community.
Which part of the research did you find personally to be the most exciting?
Observing the night sky with the best telescopes of the world.
Stefano would like to credit John Landstreet for his work on this study. John was a member of the team that discovered the first magnetic white dwarf in 1970. In the early 1970s, John discovered many more magnetic white dwarfs, before moving to the study of the magnetism of other kinds of stars (mainly Ap/Bp). A few years ago, he came back to actively study magnetic white dwarfs, and together he and Stefano have been working together intensively.
About Stefano Bagnulo
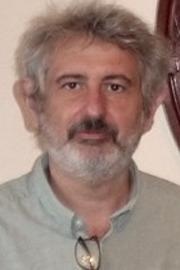
Stefano received a “Laurea in Fisica” at the University of Florence, Italy, then a PhD at Queen’s University Belfast. After three years of post-doctoral research in Vienna, he moved to work for ESO at the Very Large Telescope in Chile, first as a fellow, then as a staff Astronomer of the Science Operations Team. Since 2007 he has been a staff astronomer at the Armagh Observatory and Planetarium, UK.
Disclaimer: The views expressed here are those of the interviewee and do not necessarily represent the views of AZoM.com Limited (T/A) AZoNetwork, the owner and operator of this website. This disclaimer forms part of the Terms and Conditions of use of this website.