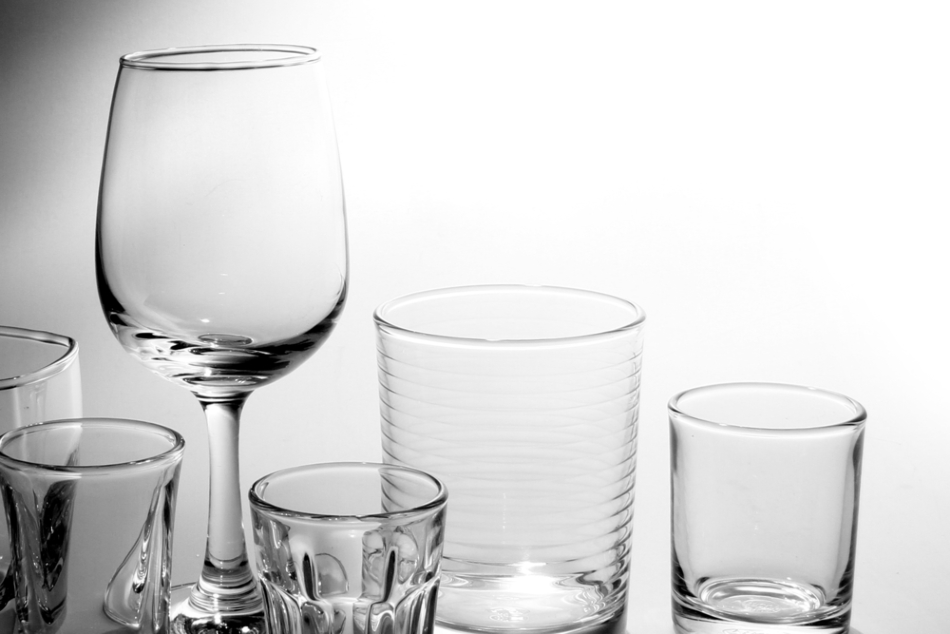
Image Credit: taffpixture/Shutterstock.com
Amorphous solids do not possess a clearly defined shape or form. Unlike crystalline solids (such as sodium chloride, ice and quartz), amorphous materials do not possess distinct melting and boiling point characteristics, regular geometric shapes, and long-range internal order. Experimental evidence suggests that most amorphous solids have some short range order (SRO) on the scale of several interatomic distances. This ‘hidden structure’ has been studied within the engineering and material science industry.
There is a growing interest in the use of amorphous materials in a range of advanced industrial and scientific applications, including:
- Glasses and ceramics
- Optical components and coatings
- Wear-resistant coatings
- Metallic glasses
- Anti-friction coatings
- Amorphous plastics (such as polystyrene)
Scientists are continuously developing new materials and improving the properties of existing ones.
High-Resolution Imaging and Diffraction
It is important to develop new methods for studying the structure-function relation in the amorphous matter. The short range atomic arrangements in the amorphous solids can be investigated either by high-resolution direct imaging techniques such as high-resolution transmission electron microscopy (HRTEM), scanning transmission electron microscopy (STEM), or by a variety of scattering techniques, with a particular focus on X-ray, neutron, and electron diffraction (ED).
High Scattering Cross-Section of the Electrons
Among the diffraction techniques, ED has a significant advantage, since the interaction between the electrons and the atoms of the amorphous sample occurs through the Coulomb forces (unlike X-rays and neutrons that interact with the electron cloud and the nuclei of the atoms, respectively).
Due to this difference, the scattering cross-section (a measure of the scattering capability of an isolated atom) for electrons is 103–104 times larger than that of X-rays and neutrons. This is especially beneficial when the sample is composed of relatively light particles (organic amorphous solids).
Quantum Particles
Like all quantum particles, the electrons (as well as the neutrons and the photons) have a characteristic wavelength that depends on their energy. The wavelength of 200 keV electrons, a typical acceleration voltage for conventional transmission electron microscopy (TEM), is 0.00251 nm (1 nm = 10-9 m), which is much smaller than that of X-rays (0.071 nm - 0.154 nm for a typical laboratory source) and neutrons (0.1 nm – 0.2 nm for thermal neutrons).
Due to their short wavelength and intense interaction with the sample material, the high-energy electron diffraction allows faster data collection at much larger scattering angles (corresponding to smaller length scales). The electron beams can be focused to an extremely fine spot size (around 1 nm or less in diameter).
Electron Diffraction of Thin Amorphous Samples
A significant drawback of the ED technique is the multiple scattering of the probing electron beam when passing through thicker specimens. This requires more elaborate and demanding sample preparation to reduce the specimen thickness down to a few nanometres (and even down to a single atomic layer).
Statistical Description of the Short Range Order
The lack of a repeatable structural unit in the amorphous materials means that it is impossible to determine the exact atomic composition at a specified location in the specimen.
The structural order of such material is described statistically by the Pair Distribution Function (PDF), which is the probability of finding an atom at a certain distance from a reference atom. It is also described using the Radial Distribution Function (RDF), which represents the average number of atoms at a given distance from any other reference atom.
These two statistical functions can be derived from the diffraction pattern of all the electrons passing through the sample (the total scattering intensity). For amorphous materials, the diffraction patterns consist of diffused rings.
Combining Experimental Data and Theoretical Models
Although the structural data reconstructed from one-dimensional RDF usually lack information about the local atomic arrangements, this statistical description of the arrangements can be used to test the consistency of three-dimensional structural models obtained using molecular dynamics simulation. By combining these two approaches, it is possible to refine structural models against diffraction data and to develop structural models that are not only in agreement with the data but are also physically reasonable.
Probing the Short Range Order with Sub-Nanometer Electron Beam
For better anatomical accuracy, the ED technique should be able to probe the atomic arrangements at the length-scale of a few inter-atomic distances. Combining modern STEM instruments with energy filters for the diffracted electrons allows a focused electron beam with a diameter of around 1 nm in diameter to be used for the observation of distinct diffraction patterns from individual atomic clusters.
However, such beam size is too large to acquire local (at nearest-neighbour length-scales) structural information that is essential for the understanding of the SRO of the amorphous solids.
Recent developments in the STEM-focusing optics allowed the development of the angstrom-beam electron diffraction (ABED) technique, where a nearly-parallel-focused electron beam with a diameter of 0.36 nm (or 3.6 angstroms, 1 angstrom = 10-10 m) is used to probe the specimen. This dramatically reduces the specimen volume interrogated by the electron beam (down to a few tens of atoms) and provides very local structural information about the atomic structure of the amorphous materials.
Industrial laboratories and production facilities, in addition to universities and research centers, have widely adopted the electron microscopy as a primary analytical approach. This makes TEM-based diffraction techniques, such as ABED, essential tools for material research and development.
References and Further Reading
A. Hirata et al. (2011) Direct observation of local atomic order in a metallic glass, Nat. Mater. 10, 28-33. Available at: https://doi.org/10.1038/nmat2897
D. J. H. Cockayne et al. (2010) The technique of RDF of nanovolumes using electron diffraction, J. Phys.: Conf. Ser. 241, 012006. Available at: https://doi.org/10.1088/1742-6596/241/1/012006
A. Hirata and M. Chen. (2014) Angstrom-beam electron diffraction of amorphous materials J. Non-Cryst. Solids. 383, 52-58. Available at: https://doi.org/10.1016/j.jnoncrysol.2013.03.010
D. J. H. (2007) The Study of Nanovolumes of Amorphous Materials Using Electron Scattering, Annu. Rev. Mater. Res. 37, 159–87. Available at: https://doi.org/10.1146/annurev.matsci.35.082803.103337
Disclaimer: The views expressed here are those of the author expressed in their private capacity and do not necessarily represent the views of AZoM.com Limited T/A AZoNetwork the owner and operator of this website. This disclaimer forms part of the Terms and conditions of use of this website.