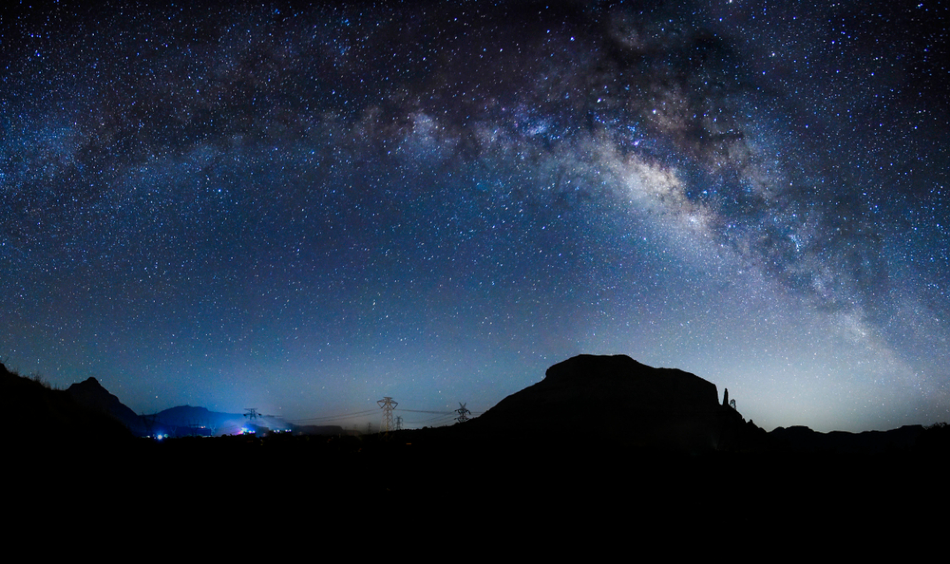
Image Credit: ABHIJIT CHABUKSWAR/Shutterstock.com
Without the counter-intuitive nature of quantum tunneling and the randomness at its heart, the stars themselves could not shine and life would not exist.
Quantum physics is often described as a mysterious and misunderstood aspect of the natural world, despite a century of research and experimentation stripping back much of its ambiguity, leaving physicists with a well-understood, albeit counter-intuitive, discipline.
Our understanding of quantum phenomena has advanced to such a stage that we now utilize it effectively in our everyday lives. From banking and communication to the future promise of quantum computing, much of the infrastructure that surrounds us is built on phenomena that once troubled Einstein so much he spent many years at the end of his life attempting to prove it incomplete.
However, quantum physics and its counter-intuitive nature run much deeper into our existence than just providing a platform for novel technologies. Were it not for one particular quantum phenomena, the stars themselves would not shine and life itself could not exist, leaving the universe a cold, dark expanse. It is the concept of ‘quantum tunneling’ that allows the stars to shine.
What Would Quantum Tunneling Look Like in Classical Physics?
Quantum tunneling is when a particle escapes a potential barrier that it does not possess the energy to clear. To explain this further, let’s consider what it would look like in the everyday, macroscopic world that surrounds us.
Imagine standing by a tall wall and throwing a ball in an attempt to pass it to the other side. Our understanding of physics tells us that to overcome the gravitational demand of reaching higher points on the wall, we must put increasing amounts of kinetic energy into the ball to increase its momentum. If you cannot put enough energy into the ball, then it is stuck on your side of the wall in a ‘bound state.’
Imagine now that the rules of quantum physics applied to the real world. You toss the ball in what you think is a futile attempt to clear the wall and reach your friend. You know from numerous tries that there is no way that you put in enough kinetic energy to reach the top of the wall. Suddenly, your friend shouts from the other side of the wall: “Got it!”
You’re perplexed as to what could have possibly happened. You assume that despite not putting enough energy into the ball to clear the wall, you somehow put in enough to punch a hole in the wall.
After a thorough investigation of the wall, you and your friend confirm there is no damage and no ‘holes’ in the wall through which the ball could have slipped. Eventually, you conclude that the ball has somehow ‘leaked’ through the wall.
This is the phenomenon we call ‘quantum tunneling.’ It occurs when a particle in a bound state is suddenly found to escape its confinement despite not possessing enough energy to clear the potential barrier ‘walls.’
The big question is why does this happen in the realm of quantum mechanics and not in our everyday world governed by classical physics? It turns out that it is a factor of one of quantum physics’ governing rules: the Heisenberg uncertainty principle.
A Certain Uncertainty
Even though there are various classes of the Heisenberg uncertainty principle that relate to the communicability of operators that form the mathematical basis of quantum physics, perhaps the most common and easiest to understand is that which relates the values of a system’s momentum to its position.
The principle states that the more precisely we know a particle’s momentum, the less information we can have about its position, and vice versa. Using our ball analogy again, the consequence of this is that if we know the amount of energy we have put into the ball, then we know to a good level of accuracy its momentum.
This means there must be a good level of uncertainty to its position, as we cannot say for sure the ball has remained on our side of the wall. There must be a small probability - which diminishes the taller or thicker the wall gets, and thus as the energy demand to clear it increases — that the ball is on the other side of the wall.
In a quantum mechanical system with a particle-bound in a potential well, there is a small probability that the particle has escaped despite not possessing enough energy to do so. Particles in the quantum world are described by wave-like behavior and mitigated by probabilistic mathematics, meaning there is always a small chance that a particle may overcome a seemingly insurmountable obstacle to be found somewhere it really does not belong.
This may well lead to the question that if this probability is so small, how can it result in the tremendous power output of the stars?
Back to the Beginning: Quantum Tunneling in Stars
The major hindrance towards nuclear fusion within the stars is the fact that the initial chain reaction must be kick-started by the merger of two protons. To come close enough to each other to fuse, these protons must overcome the electric repulsion of their mutual positive charges. Even with the massive velocities that particles within the violent conditions of a star’s core possess, it is still not enough to overcome this Coulomb barrier.
This means quantum tunneling must come to the rescue. But, even with this effect, does the low probability of particle tunneling not mean that very few proton pairs would initiate fusion?
For example, the Sun produces a continuous 4x10²⁶ Watts, which requires 4x10³⁸ protons to overcome the Coulomb barrier and fuse every second. Fortunately, this last leap of logic required is provided by the law of large numbers. With around 10⁵⁶ protons in the Sun’s core — even an extremely rare event can occur frequently.
It is fair to say that life itself — not possible without the power provided by the Sun — owes everything to the non-intuitive and random nature of quantum physics.
References and Further Reading
Bolton. J, Lambourne. R, (2007), Wave Mechanics, Open University.
Griffiths. D, (2017), Introduction to Quantum Mechanics, Cambridge University Press.
Disclaimer: The views expressed here are those of the author expressed in their private capacity and do not necessarily represent the views of AZoM.com Limited T/A AZoNetwork the owner and operator of this website. This disclaimer forms part of the Terms and conditions of use of this website.