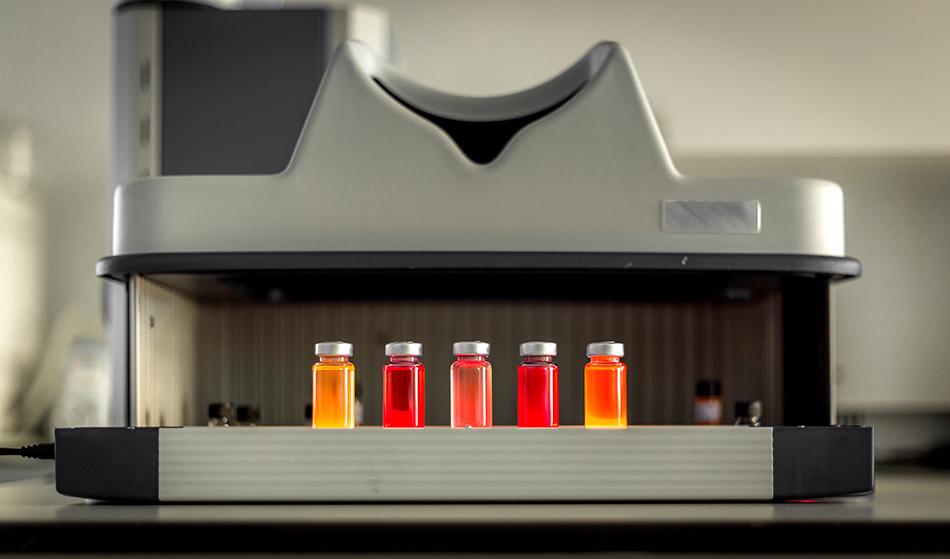
Tayfun Ruzgar/Shutterstock
Quantum dots have long been considered one of the most promising materials for use in next-generation solar cells. With the potential for significantly increased efficiencies at a fraction of the price of current devices, quantum dots could help revolutionize photovoltaics in a world where the demand for renewable energy has never been greater.
Quantum dots are extremely small semiconductor particles, with diameters only a couple of nanometers in length. Quantum confinement of electrons in these semiconductor crystals results in discrete energy levels, a characteristic that has led many to describe quantum dots as artificial atoms.
Varying the size of quantum dots allows us to adjust the spacing of their energy levels or tune their bandgap (the energy required to liberate an electron) to suit our needs. Tuneable bandgaps are what make quantum dots so desirable for solar cells.
Current cells utilize a conventional semiconductor, typically silicon, as the light absorbing material. Here, an electron-hole pair (an exciton) is produced and swept away by the cell’s electric field, producing a current. However, to produce an exciton, the energy of an incident photon must be equal to the bandgap of the semiconductor (for silicon this is 1.1 eV).
Photons with insufficient energy will not be absorbed, and those with excess energy will lose it through collisions within the cell, heating the device. Both processes lower the effectiveness of single-layer solar cells, which have a theoretical conversion efficiency limit of 33%. This can be increased by stacking semiconductors with different bandgaps; however, the cost of such multi-layer cells limits their use to space where the power-to-weight ratio justifies the expense.
Quantum dots have the potential to be a more efficient, cost-effective alternative to current captivating photovoltaic materials. The cost of fabricating various quantum dots is significantly lower than altering the bandgaps of conventional semiconductors. Additionally, photon energies in the far infrared, previously unobtainable using traditional solar cells, can be captured using lead sulfide quantum dots.
Given about half of the solar energy reaching the earth’s surface is in the infrared region, the ability to utilize these photons significantly increases the conversion efficiency of quantum dot solar cells (QDSCs), which, in theory, could reach 66%. The ability to produce several electrons from one high energy photon (a phenomenon known as multiple exciton generation) also contributes to the increased efficiency of QDSCs.
On top of this, the manufacture of QDSCs requires considerably less energy than current devices, further lowering their cost, and the finished product is comparatively lightweight, resulting in a higher power-to-weight ratio.
A major problem facing the commercialization of QDSCs is the inherent instability of quantum dots when exposed to air. Oxidation as a result of exposure to the atmosphere can alter the intrinsic properties of quantum dots, increasing the bandgap energy and thus reducing the absorption of light.
Quantum dot defects can also result in surface traps which greatly increase the probability of exciton recombination, resulting in a decrease in energy output. Current research is centered on improving quantum dot stability in ambient conditions, with methods including halide ion treatments and the introduction of chalcogenide outer shells and is yielding promising results. The toxicity of quantum dots containing elements such as lead or cadmium is also a concern; however, progress has been made utilizing polymer shells and alternative non-toxic materials.
It is clearer than ever before that there is an urgent need to better utilize the 120,000 TW of power provided by the sun, and with the solar power industry expected to grow tenfold by the year 2030, advancements in photovoltaics are eagerly anticipated. Research into exploiting the optical properties of quantum dots has produced encouraging results, and, although not yet commercially viable, QDSCs could help pave the way to a more sustainable future.
Additional reading:
Disclaimer: The views expressed here are those of the author expressed in their private capacity and do not necessarily represent the views of AZoM.com Limited T/A AZoNetwork the owner and operator of this website. This disclaimer forms part of the Terms and conditions of use of this website.