AZoQuantum talks with Dusan Saranec about his research that looks at using twisted neutrons to characterize materials and verify the fundamentals of physics.
Please could you introduce yourself and your research background?
My name is Dusan Sarenac and I am currently a Senior Technical Lead of Transformative Quantum Technologies at the University of Waterloo. I am an experimental quantum physicist with a broad background and highly interdisciplinary experience in the fields of quantum information science, condensed matter, photonics, matter waves, and medicine and health. My main research interests are in developing quantum technologies and my current role is to accelerate the development and deployment of impactful quantum devices. I hold a Bachelor of Applied Science in Nanotechnology Engineering and a Ph.D. in Physics (Quantum Information).
My graduate research heavily focused on the development of neutron optics and neutron interferometry techniques. Along with my colleague Dmitry Pushin and supervisor David Cory, we had developed several innovative techniques for neutron interferometry, devices for generating spin-orbit beams, as well as neutron holography. In addition, we demonstrated several theoretical and experimental neutron implementations that were based on quantum information science. As a continuation of that work, we are currently developing methods and devices for generating and characterizing neutron waves whose wavefronts are shaped to enable the manifestation of special properties.
What are neutrons and why are they a better investigative tool than protons or electrons?
Neutrons are subatomic particles found inside the nucleus of most atoms. Typical atoms consist of a central nucleus (composed of neutrons and protons) that is surrounded by negatively charged electrons. When it comes to exploratory endeavors, one has to choose the appropriate probe depending on which property one wishes to investigate. For example, if one wishes to characterize the surface properties of a material then one needs a probe such as light or electrons that strongly interacts with the surface. Neutrons on the other hand are great at probing bulk properties as they do not possess an electric charge and easily penetrate materials. They essentially serve as a complementary probe of materials and nature. There are many instances where the use of neutrons is much more convenient and powerful.
How can neutrons be used to characterize materials and verify the fundamentals of physics?
Whereas the bound neutrons in atoms are stable, the lifetime of an unbound neutron (such as the free neutrons produced in nuclear reactors) is around 15 min. Research Reactors are nuclear reactors that primarily serve as a neutron source for scientific research. The produced neutrons can be guided, collimated, and directed to various beamlines where they are inputted into various neutron setups. Neutrons are a convenient probe of materials and fundamental physics due to their relatively large mass, electric neutrality, and sub-nanometer-sized wavelengths. Some hallmark experiments include the first demonstration of gravity on a quantum particle, probing of dark energy/fifth forces, observing the 4π symmetry of spinor rotation, and characterization of fuel cells.
How does this relate to quantum materials?
Spin textures and spin topologies are the emergent properties of a material in which the material’s spin is non-trivially coupled with its other dynamical degrees of freedom, such as the position, momentum, or orbital angular momentum (OAM). These intricate correlations which in essence manifest entanglement between the spin and the other degrees of freedom are responsible for a rich variety of thermodynamic phases of matter. For example, Skyrmions and Merons may materialize, thus providing a basic platform for future applications in spintronics. While conventional probes provide indirect signatures of topological excitations, spin-textured beams of neutrons with specific spin-orbit couplings (e.g., those with definite states of OAM) are strongly desired because they may act as direct probes of the target’s topology.
Can you explain how structured waves of light, electrons, and atoms are important as scientific tools?
Interest in structured waves of light and matter, and orbital angular momentum (OAM) waves in particular, is demonstrated by several high-profile review articles published during the past year. Studies of optical orbital angular momentum (OAM) have blossomed since the early 1990s and are now encompassed in a larger framework of structured waves of light and matter. OAM has been induced in beams of light, electrons, atoms, and molecules.
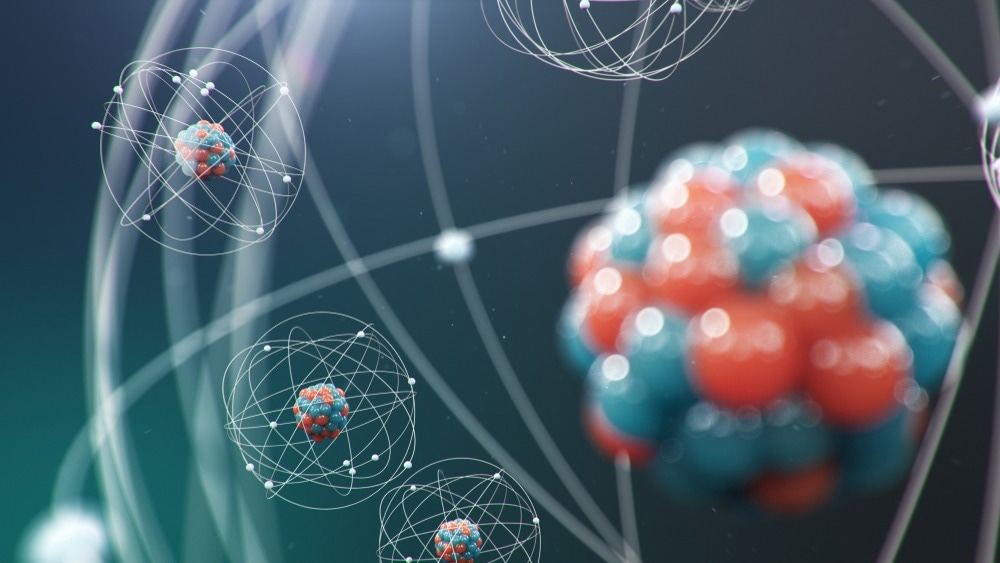
Image Credit: Rost9/Shutterstock.com
Photonic OAM has demonstrated usefulness in edge-detection microscopy, quantum information processing protocols, encoding and multiplexing of communications, and optical manipulation of matter. Electron OAM beams have found applications in the characterization of nanoscale magnetic fields in materials and the exploration of magnetic monopoles.
What have been some of the problems that have stopped these techniques from being employed in neutron science?
There are several problems when dealing with neutrons that are typically not encountered in standard optics. The neutron flux is extremely small compared to a typical laser. One can buy handheld lasers that emit more photons than the neutron numbers we typically work with in cutting-edge experiments. In addition, the standard optics components such as lasers that emit collimated and coherent light do not exist for neutrons. Lastly, the detection methods for neutrons are poor compared to typical optical cameras. For example, the pixel size of the neutron camera we used in our recent experiment was around 5mm by 5mm.
Why does working with neutrons require a fresh approach?
Neutrons’ weak interaction with common materials makes them difficult to control and manipulate. Many components that exist for optical setups are not yet available for neutron setups. For example, the basic optimal element, a lens, is not practical in neutron setups. Therefore, it is a challenge to develop and fabricate devices that control neutrons in a desired manner. In the given case the challenge was inducing an azimuthally dependent phase shift that carries orbital angular momentum.
What is orbital angular momentum and how does it relate to these techniques? Can you explain why you turned to ‘twisted’ OAMs to tackle these problems?
Structured waves refer to the generation and application of custom wave fields. A general class of structured wave functions is indexed by orbital angular momentum (OAM), in which the wave function varies azimuthally. The OAM states have a “helical” or “twisted” wavefront, and they have been shown to manifest unique sets of selection rules and scattering cross sections when interacting with matter. The challenge addressed in this work was inducing this helical wavefront over the individual neutrons.
Can you describe your approach to creating ‘twisted neutrons’?
We have experimentally demonstrated a method to generate and characterize neutron helical waves that are dominated by a well-defined OAM value. The experimental demonstration used devices that consisted of a 2D array of fork dislocation phase gratings, with a pattern area of 0.5 cm by 0.5 cm which amounts to 6,250,000 individual fork dislocation phase gratings contributing to the observed signal. Each grating possessed a period of 120 nm, height 450 nm, and was separated by 1 µm on each side from the other gratings. The topological charge (q) of the grating sets the OAM values carried by the diffraction orders. To detect generated OAM states we mapped out the momentum distribution at a Small Angle Neutron Scattering (SANS) beamline where we observed the doughnut-like OAM signal from the diffraction orders.
What makes this research a first in materials science?
Neutrons have been popular in the experimental verification of fundamental physics, using the three easily accessible degrees of freedom: spin, path, and energy. In these experiments, our group has enabled the use of orbital angular momentum in neutron beams, which will essentially provide an additional quantized degree of freedom. This opens up the doors for the next generation of high-impact neutron experiments.
What are some of the applications of this breakthrough? / What is next for this research and what are you and the team hoping to improve?
The presented method opens the door for the implementation of other structured wave techniques with neutrons, such as the generation of “self-accelerating” Airy beams and the “nondiffractive” Bessel beams. These beams have a “self-healing” property, as they can reform after being partially obstructed. Furthermore, the convenient integration with a SANS beamline provides access to the far field where the helical beams with specific OAM values are separated in the form of diffraction orders. This enables studies of interactions between neutron’s helical degree of freedom and scattering from materials. For example, placing the array of fork dislocation phase gratings before a topological sample and postselecting the analysis on individual diffraction orders allow for direct study of scattering properties from neutron helical waves.
What surprised you the most about your findings?
One of the biggest surprises for me was the realization that our experiment additionally provides a direct measure of the transverse size of each neutron, and that we measured it to be over 10cm in size. There is an ongoing debate in neutron science about the measurements of the neutron transverse wavefunction, which is essentially the spatial extent that a single neutron occupies. The realization that our experiment was a direct measurement of neutron coherence was a pleasant surprise.
Where can readers find more information?
Our articles on neutron orbital angular momentum and structured waves are open access. I would recommend the following:
https://www.science.org/doi/full/10.1126/sciadv.add2002
https://www.pnas.org/doi/full/10.1073/pnas.1906861116
https://www.nature.com/articles/nature15265
https://opg.optica.org/oe/fulltext.cfm?uri=oe-24-20-22528&id=350260
About Dusan Sarenac
I am currently a Senior Technical Lead of Transformative Quantum Technologies at the University of Waterloo, and my current role is to accelerate the development and deployment of impactful quantum devices. My career highlights include being a part of the group to achieve: the demonstration of neutron phase-grating moiré interferometers, neutron holography with macroscopic objects, extension of structured waves to visual science and human perception, the development of neutron tomography methods for material characterization, the demonstration of neutron orbit and spin-orbit beams, and the extension of these methods to optics and single photons. Lastly, I contributed to the development of a new polarized neutron interferometry facility at the National Institute of Standards and Technology Center for Neutron Research that is dedicated to quantum information related experiments.
Interview Questions Assisted by Robert Lea
Disclaimer: The views expressed here are those of the interviewee and do not necessarily represent the views of AZoM.com Limited (T/A) AZoNetwork, the owner and operator of this website. This disclaimer forms part of the Terms and Conditions of use of this website.