AZoQuantum spoke with Dr. Sai Kiran Rajendran about new research on the use of cuprous oxide crystals of high purity able to demonstrate Rydberg exciton-polaritons of quantum numbers as large as 6.
Could you please introduce yourself and your professional career?
I am Dr. Sai Kiran Rajendran and am currently working as a research associate at the Institute of Photonics, University of Strathclyde, UK. From 2015 to 2021, I worked as a research fellow at the School of Physics and Astronomy, University of St Andrews, UK, first at the Organic Semiconductor Centre, and later at the Quantum Light-Matter group.
From 2010-2015, I worked at the Ultrafast spectroscopy group at Politecnico di Milano, Italy, and obtained a Ph.D. degree in 2014. I graduated in 2009 with a Masters degree in Physics from Sri Sathya Sai Institute of Higher Learning, India.
The results on Rydberg polaritons were obtained during the period between 2019 and 2021, as part of the EPSRC project 'Rydberg Polaritons in Cu2O microcavities', led by Dr. Hamid Ohadi, Quantum Light-Matter group, at the University of St Andrews.
Could you please explain what Rydberg polaritons and hybrid particles of light are?
An exciton is a pair of negatively charged electrons and a positively charged hole in a semiconductor material bound by Coulomb attraction. In semiconductor materials, the energy of a series of excitons can be described by a Rydberg formula. Each level of the excitons in the series is represented by a positive integer quantum number. High quantum number excitons are called Rydberg excitons.
Light can be temporarily trapped in photonic cavities that consist of highly reflective mirrors. Excitons that demonstrate strong absorption of light and possess a long lifetime can be strongly coupled to light by sandwiching the semiconductor material between highly reflective mirrors. Strong coupling leads to the formation of hybrid light-matter quasiparticles called polaritons that exhibit a mixture of light-matter properties - for example, the effective mass of a polariton is the weighted average of its fractional light and matter components.1,2 When Rydberg excitons are strongly coupled to light, they form Rydberg exciton-polaritons.
Your research is facilitated by the discovery and use of a cuprous oxide gemstone from Namibia. What is unique about this gemstone?
The gemstone cuprite is crystalline cuprous oxide. This stone can be polished to thin slabs of optical quality that can be used for spectroscopic studies. While cuprous oxide can also be synthesized, natural cuprous oxide crystals of high purity are able to demonstrate Rydberg excitons of quantum numbers as large as 30.3,4 Such large quantum number excitons have not been observed in any other material.
How are Rydberg polaritons created and what was special about how your team went about achieving this?
Highly reflective mirrors called distributed Bragg reflectors were fabricated using a sputter deposition technique. These mirrors were designed to trap light for about 2000 reflections between them with a lifetime that matched that of Rydberg excitons in cuprous oxide. A crystalline stone of cuprous oxide was thinned to around 30-micrometer thickness, and its surfaces were polished to sub-micron roughness.
This thin slab was sandwiched between the two mirrors, cooled down to 4 K temperature, and light was transmitted through the microcavity to strongly couple Rydberg excitons up to quantum number 6 to light trapped in the microcavity.5 So far, all studies of strongly coupled excitons in different materials to light were of quantum number 1 or 2 only. Our result is a significant improvement because the properties of Rydberg excitons scale exponentially with their quantum number.6
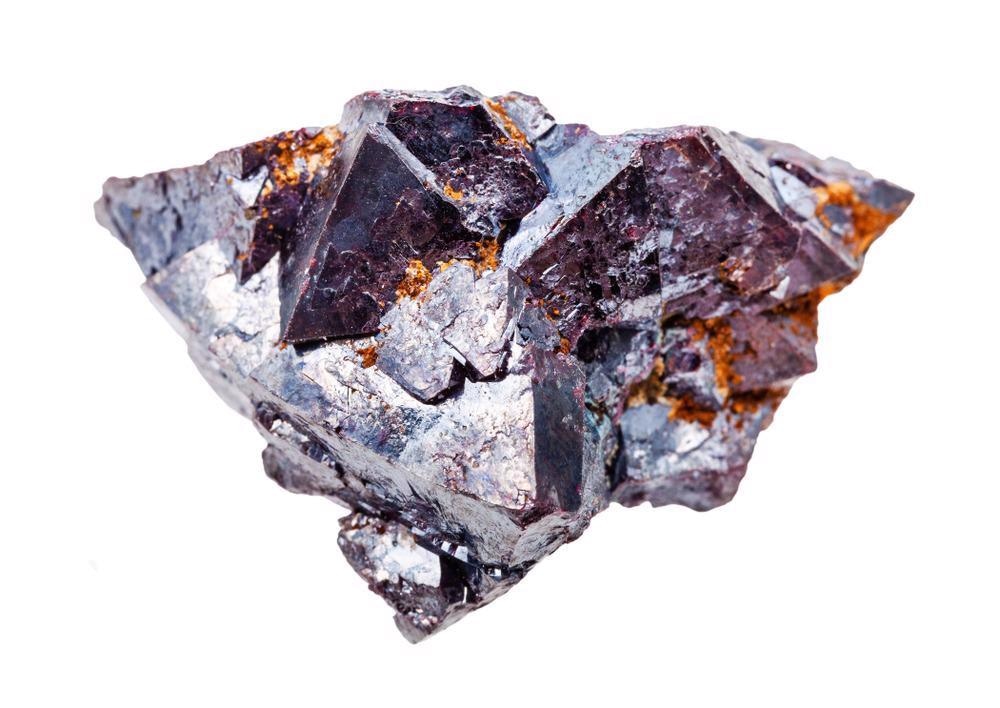
Image Credit: vvoe/Shutterstock.com
How is the interaction of light and matter seen in Rydberg polaritons useful practically?
By strong coupling light to Rydberg excitons, quantum non-linear properties of light can be achieved. For example, Rydberg-mediated electromagnetically induced transparency can be realized, which can then be used for applications as single-photon switches and quantum logic gates.7
Can you explain how this phenomenon can be applied to quantum computers?
Rydberg excitons demonstrate a phenomenon called Rydberg blockade, which enables Rydberg excitons to act as a controlled-NOT gate. This gate can then be expanded to other quantum logical operations to manipulate qubits.8 The higher the quantum number of the exciton, the larger its blockade effect. Rydberg polaritons can then enable the transmission of such qubits through photons or its light component.
What is the difference between quantum simulators and a “regular” quantum computer?
A quantum simulator is a quantum system that simulates/imitates the behavior of another quantum system. It enables us to understand the physical and chemical properties of quantum materials, such as the many-body problem. A quantum computer, on the other hand, uses a quantum system to perform calculations that are too complex for a classical computer, such as finding prime factors of large numbers.9
What applications would quantum simulators be particularly useful for?
Quantum simulators10 can be used to understand the behavior of atomic particles at the microscopic level through the many-body problem. A macroscopic solution does not take into consideration the individual behavior of each atom in a large crystal and is therefore easy to compute, but only approximates the behavior of the system. By using a quantum simulator, it is possible to understand the properties of a system at the atomic level.
For example, the Bose-Hubbard model11 describes a system of many particles interacting with each other. A system described by this model behaves as an insulator when the interaction strength is large, or as a superfluid when the hopping capacity is large. With Rydberg polaritons, it will be possible to tune the interaction strength to a much larger degree than with just light/photons. Rydberg exciton-polaritons in cuprous oxide will make it much easier to tune the interaction strength than is currently possible with Rydberg polaritons in neutral atoms.
During the course of your research into Rydberg polaritons, was there anything that shocked or surprised you and the team?
In the current age of highly complex instrumentation and technology, where scientific studies use intensive purifying and manufacturing techniques, it was surprising to see the level of advanced physics that can be studied using a rudimentary stone.
What are the next steps for this research?
The next steps are to obtain a Rydberg blockade of polaritons in cuprous oxide and build a quantum circuit. Thereafter, we will focus on the control and scalability of such circuits for quantum simulation.
How far are we from realizing quantum simulators, and what hurdles remain in the creation of this technology?
Quantum simulation has been realized using different systems consisting of neutral atoms, trapped ions, atomic cavity arrays, electron spins in quantum dots, superconducting circuits, photons using linear optics, and nuclear spins. Each of these systems possesses different strengths and weaknesses with respect to control and scalability. Further studies are ongoing to address these issues.12 We are excited to explore the prospects of Rydberg exciton-polaritons for quantum simulation.
More Interviews on AZoQuantum: How Future Information Transfer Could Be Revolutionized By Skyrmions
Where can readers find more information?
- Khitrova, G., Gibbs, H. M., Kira, M., Koch, S. W. & Scherer, A. Vacuum Rabi splitting in semiconductors. Nat. Phys. 2, 81–90 (2006).
- Sanvitto, D. & Kéna-Cohen, S. The road towards polaritonic devices. Nat. Mater. 15, 1061–1073 (2016).
- Kazimierczuk, T., Fröhlich, D., Scheel, S., Stolz, H. & Bayer, M. Giant Rydberg excitons in the copper oxide Cu2O. Nature 514, 343–347 (2014).
- Versteegh, M. A. M. et al. Giant Rydberg excitons in Cu2O probed by photoluminescence excitation spectroscopy. Phys. Rev. B 104, 245206 (2021).
- Orfanakis, K. et al. Rydberg exciton–polaritons in a Cu2O microcavity. Nat. Mater. (2022) doi:10.1038/s41563-022-01230-4.
- Heckötter, J. et al. Scaling laws of Rydberg excitons. Phys. Rev. B 96, 125142 (2017).
- Peyronel, T. et al. Quantum nonlinear optics with single photons enabled by strongly interacting atoms. Nature 488, 57–60 (2012).
- Saffman, M., Walker, T. G. & Mølmer, K. Quantum information with Rydberg atoms. Rev. Mod. Phys. 82, 2313–2363 (2010).
- Buluta, I. & Nori, F. Quantum Simulators. Science 326, 108–111 (2009).
- Cirac, J. I. & Zoller, P. Goals and opportunities in quantum simulation. Nat. Phys. 8, 264–266 (2012).
- Hartmann, M. J., Brandão, F. G. S. L. & Plenio, M. B. Quantum many-body phenomena in coupled cavity arrays. Laser Photonics Rev. 2, 527–556 (2008).
- Georgescu, I. M., Ashhab, S. & Nori, F. Quantum simulation. Rev. Mod. Phys. 86, 153–185 (2014).
About Dr. Sai Kiran Rajendran
I am currently working as a research associate at the Institute of Photonics, University of Strathclyde, Glasgow, on a project that aims to realize quantum enhanced microscopy by using entangled photons. I worked on Rydberg polaritons for applications in quantum technologies between 2019-2021 at Quantum Light-Matter group, School of Physics & Astronomy, University of St Andrews, . Earlier in my research career I investigated the photo-physics of organic semiconductors and polaritons using ultrafast spectroscopy (2010-2015) and low threshold room temperature polariton lasing (2015-2019). In 2020 I was awarded a research incentive grant from the Carnegie Trust for the Universities of Scotland to investigate quantum confined Rydberg excitons. I obtained my PhD degree in 2014 supported by funding from an EU ITN-FP7 Marie Curie project on hybrid organic-inorganic nanostructures for photonics and optoelectronics.
Interview questions provided by Robert Lea.
Disclaimer: The views expressed here are those of the interviewee and do not necessarily represent the views of AZoM.com Limited (T/A) AZoNetwork, the owner and operator of this website. This disclaimer forms part of the Terms and Conditions of use of this website.