Superconducting qubits form an attractive platform to build scalable quantum computers, yet the traditional fabrication methods yield qubit assemblies that are substantially larger than the semiconductor chips found in classical computers.
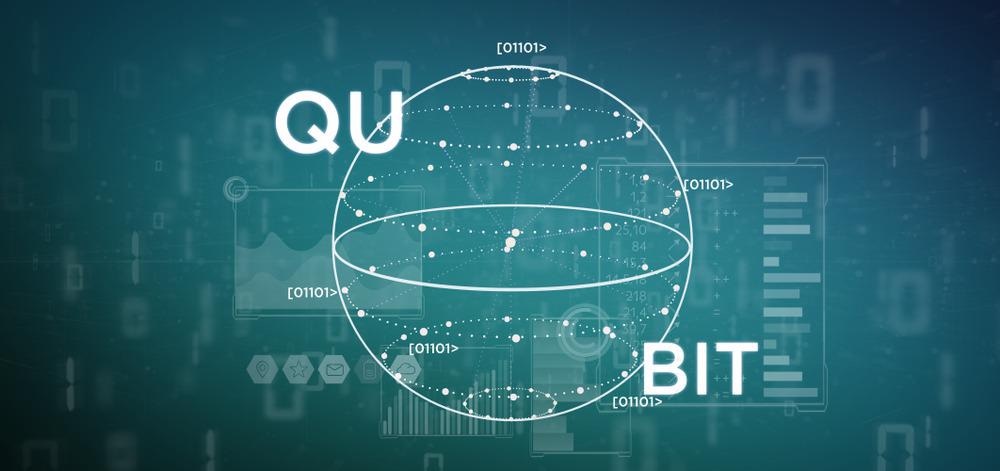
Image Credit: Production Perig/Shutterstock.com
To shrink the qubit size down, researchers at Columbia University and Raytheon BBN Technologies employed atomically-thin 2D materials to manufacture ultrasmall capacitors needed for the superconducting qubits. The resulting two-qubit demonstrator chip was three orders of magnitude smaller than those made using conventional materials.
Scientists hope that achieving fault-tolerant quantum computation could revolutionize many areas of scientific research, such as simulation of climate models and discovering new medicines, which require enormous amounts of calculations. In theory, quantum computing could solve such problems faster and more efficiently than the existing computer technology.
The classical computers manipulate long series of bits that can be set to 0 or 1. In contrast, a quantum computer operates by manipulating quantum bits, or qubits, that can exist in both states (0 and 1) simultaneously.
Qubits – A Key Component of Quantum Computers
There are multiple ways of building a qubit. Creating nitrogen-vacancy centers (N-V centers) as point defects in diamond substrates results in the so-called spin-qubit. To operate, the spin-qubits require high-efficiency optical systems, such as immersion optics or all-diamond micro-optics, which hinders the scaling of spin-based quantum computers at present.
Topological qubits offer a great promise to make quantum computing more robust against outside interference as their intrinsic properties enable a stable qubit state regardless of the external interference. However, the topological qubits proved extremely difficult to create so far.
Because of their ease to fabricate, the superconducting qubits (also known as transom qubits) are the most widely-used ones and currently represent the industry standard in quantum computing. Some of the largest quantum computers built by IBM and Google utilize transom qubits in their architectures.
Why Qubit Miniaturization Is a Challenge?
A superconducting qubit is an oscillator consisting of a Josephson junction (a device made by sandwiching a thin layer of a nonsuperconducting material between two layers of superconducting material) and a capacitor. The operating frequency of the oscillator cannot be too high, which requires a relatively large capacitance of the capacitor, otherwise controlling the qubit becomes difficult.
It is possible to create capacitors with large capacitance and small sizes, such as meshed combs or multilayer metal-dielectric stacks, but these configurations increase the field strength in the capacitor and the susceptibility of the qubit to decoherence due to external interference.
More from AZoQuantum: How Can Fiber Optic Cables Help Transport Quantum Keys?
To achieve large enough capacitance with simple planar electrode designs without using dielectric materials requires devices with substantial footprints. Typically, the area of the capacitor in a transom qubit is several thousand square microns, which is many orders of magnitude larger than a transistor in a computer integrated circuit.
Miniaturizing Qubits Using van der Waals 2D Materials
Recently, a research group at Columbia University in New York led by Prof. James Hone, together with colleagues from the Quantum Engineering and Computing Group at Raytheon BBN Technologies (an advanced technology research specialist company based in Princeton, USA), utilized atomically-thin 2D materials to build a superconducting qubit capacitor with a size that is a fraction of that of the existing devices.
To achieve this, the scientists sandwiched an insulating monoatomic layer of hexagonal boron nitride between two monoatomic layers of superconducting niobium diselenide. The stacked layers interacted via van der Waals forces that were sufficient to keep the stack together, while the in-plane stability of the individual 2D layers was provided by stronger covalent bonds.
The resulting van der Waals heterostructure, with a total area of 109 square microns (around 1400 times smaller than the conventional planar capacitors) and a thickness of only 35 nanometers, served as a parallel-plate capacitor for the transom qubit. The team then combined the van der Waals heterostructure capacitors with pre-patterned niobium circuits and aluminum-aluminum oxide-aluminum Josephson junctions to create a chip containing two qubits.
Record-Breaking 2D Qubits Pave the Way towards Next-Generation Quantum Computing
Prof. Hone and his colleagues characterized the qubits made with the 2D van der Waals heterostructures at temperatures close to the absolute zero and observed a quantum coherence, a phenomenon where the two qubits were becoming entangled and act as a single unit. The coherence time was short, just over 1 microsecond, compared to the typical coherence time of around 10 microseconds for conventionally-built qubits. Still, the scientists were able to manipulate and read out the quantum state of the qubit.
At the same time, a research team led by Andrew Houck, a professor of Electrical and Computer Engineering at Princeton University, took advantage of replacing the niobium with tantalum to fabricate 2D transmon qubits that exhibited record-breaking coherence times that exceeded 300 microseconds.
These first steps in exploring the use of 2D materials in quantum computing demonstrate the potential of the novel method to assemble stacks of a wide range of different 2D materials with virtually no structural limitations that can improve qubit coherence.
References and Further Reading
Antony, A., et al. (2021) Miniaturizing Transmon Qubits Using van der Waals Materials. Nano Letters 21 (23), 10122-10126. Available at: https://doi.org/10.1021/acs.nanolett.1c04160
T. E. Roth, R. Ma, W. C. Chew (2021) An Introduction to the Transmon Qubit for Electromagnetic Engineers. arXiv:2106.11352v1. Available at: https://arxiv.org/abs/2106.11352v1
Place, A.P.M., et al. (2021) New material platform for superconducting transmon qubits with coherence times exceeding 0.3 milliseconds. Nat Commun 12, 1779. Available at: https://doi.org/10.1038/s41467-021-22030-5
E. Neff (2021) Shrinking Qubits for Quantum Computing with Atom-Thin Materials. [Online] Columbia University. Available at: https://www.engineering.columbia.edu/press-release/hone-lab-superconducting-qubit-chip
Ball, P. (2021) First quantum computer to pack 100 qubits enters crowded race. Nature 599, 542. Available at: https://doi.org/10.1038/d41586-021-03476-5
Disclaimer: The views expressed here are those of the author expressed in their private capacity and do not necessarily represent the views of AZoM.com Limited T/A AZoNetwork the owner and operator of this website. This disclaimer forms part of the Terms and conditions of use of this website.