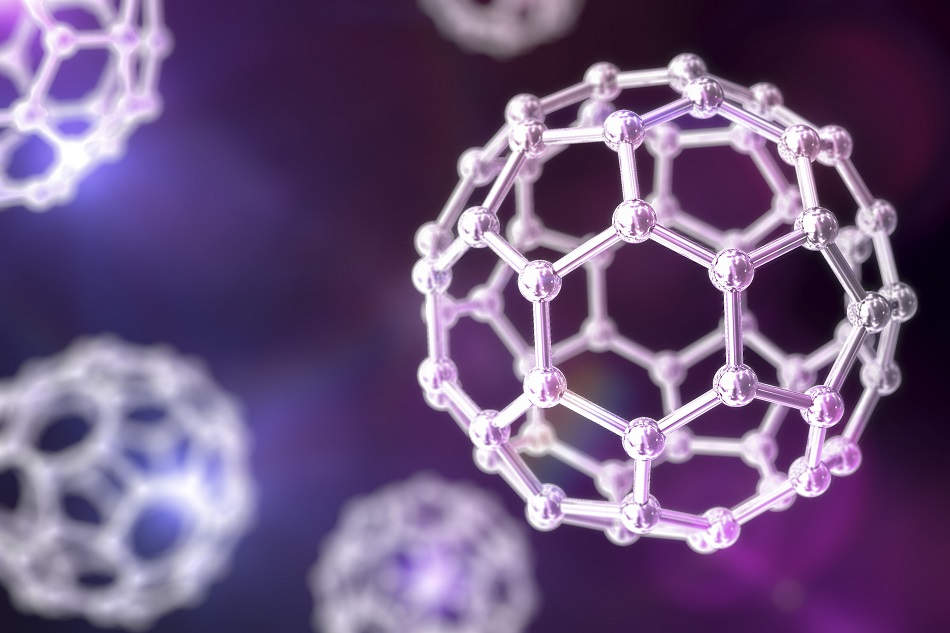
Image Credit: Kateryna Kon/Shutterstock.com
Article updated by Suzie Hall on 27/02/20
Nanoparticles refer to minuscule particles of 1 to 100 nm in size (in one dimension). Particles at the lower end of this distribution are governed by interesting quantum effects, which often make their characteristics wholly different from their macroscale counterparts. Behaviors such as their fluorescence, electrical conductivity, and chemical reactivity change as a function of their size.
They are of particular interest because they provide a "bridge" between individual atoms and bulk materials, and their unique properties have fascinated scientists for decades.
Nanoparticles at a Glance
In a bulk material, the majority of the component atoms are interior (as opposed to at the surface), and so the physical properties of the material are determined by this inner core. However, with nanoparticles, the surface layer, which is made of matter such as ions, other molecules or surfactants, becomes nonnegligible and begins to define the nanoparticles’ characteristics with as equal weighting as the core. Essentially, the relatively large surface area of nanoparticles makes them more chemically reactive and can affect their strength or electrical properties.
Nanoparticles are easily synthesized and can be configured and manipulated in a host of different ways (i.e. with different cores and surface layers) to achieve the desired outcome. Take the example of gold nanoparticles: at the macroscale, gold is well-known for its shimmering yellow color, but it appears red or purple at the nanoscale. This is due to the confined motion of the particles, which causes them to interact differently with light compared to larger gold particles. This behavior can be put to practical use, for example, to selectively accumulate in cancerous tumors, allowing for precise imaging and targeted laser destruction, while avoiding healthy cells.
The Quantum Confinement Effect
The properties of any material are essentially just the average of the quantum effects acting on those atoms. As the particle size is shrunk – eventually reaching nanosize – this averaging no longer works to describe the material’s physical properties, and we must look at each individual atom’s quantum behavior – and their interactions with one another – instead.
This effect (also known as the quantum size effect) is due to a phenomenon known as confinement and is more prevalent in nanoparticles of 10 nm or less. It is well-known that particles can be described as acting like a wave or a particle.
In a bulk material, the electrons are generally treated as wave-like and are “free” to move between atoms. As we shrink the size of a particle, the spatial extent of electron wave-function is comparable to the particle’s size, and the electron begins to “feel” the presence of particle boundaries and adjust their energy accordingly. In this way, electrons are now “confined” in quantized energy levels and the once freely-moving electrons are now restricted into these specific levels.
Materials suddenly exhibit very different properties: opaque substances such as copper become transparent; stable materials such as aluminum turn out to be combustible; solids like gold become liquid at room temperature; and insulators such as silicon become conductors.
Quantum Dots
A powerful and fascinating result of quantum effects on the nanoscale is the concept of ‘tunability’. By changing particle size, one can fine-tune a material’s property of interest - such as changing the fluorescence color - which can then be used to identify particles and label them with markers for various purposes.
Quantum dots are one of the most significant developments which exploit such quantum tunability. They are nanoparticles less than 10 nm in size, made of semiconductor materials that have fluorescent properties. Their properties are closely related to their size and shape, and they lie between those of bulk semiconductors and discrete molecules.
The Mechanics of Quantum Dots
Due to the quantization of the electrons’ energy, the dots can be easily manipulated to fluoresce at predefined wavelengths. When incident light is shone on semiconductor material, electrons are excited to a higher state and leave behind a ‘hole’. The excited electron and subsequent hole exist in a bound state – known as an exciton – attracted to one another by the electrostatic Coulomb force. After a certain (usually exceptionally short) length of time, the electron returns to its ground state, emitting energy as a photon – a particle of light. This is the principle of fluorescence.
The emitted photon’s energy is determined by the band gap energy between the highest occupied and lowest unoccupied energy levels, the confinement energies of the hole and the excited electron, and the bound energy of the exciton. Quantum dots – bound by the quantum confinement effect – are highly manipulable and, as such, they can be fine-tuned to exact fluorescing wavelengths. Quantum dots are up to a thousand times brighter and glow longer than conventional fluorescent dyes.
Smaller dots have a greater band gap and, ultimately, absorb and emit at higher wavelengths, with their light being bluer. Conversely, larger dots have a lower energy gap and absorb and emit towards the redder end of the spectrum. Size plays an important role in the synthesis of quantum dots: smaller dots suffer the effects of the quantum realm more readily and, as such, are more tuneable. Larger dots have an extended lifetime due to their shortened bandgap.
To improve the fluorescence quantum yield – essentially the “return rate” of fluorescing photons – it is possible to add a shell to a quantum dot, usually composed of a larger bandgap semiconductor material. This is thought to reduce the access of electron-hole pairs to alternative recombination pathways and improves the overall yield.
Quantum Dots in Quantum Computing
Such dots are promising for the development of solid-state quantum computers. A “qubit” is the basic unit of quantum information, equivalent to a classical bit in our modern binary systems. Quantum properties such as “spin” and “charge” can be seen as qubits, and exploited in quantum computing. Contrary to a classical system – qubits can exist in both states at once, instead of just one or the other.
Quantum dots are being investigated as “housing units” for subatomic particles such as electrons - which have inherent spin, charge and other quantum properties – to be used as qubits. These quantum dots can be placed in arrays, connected to outside electronics for control and reading of the qubit states. While most prototypes in this field are still in their nascence, quantum dots are showing as a promising solution to some of the problems encountered in quantum computing development.
Quantum Entanglement
Quantum entanglement is an intriguing property of quantum particles. The phenomenon states that two particles such as electrons, photons or atoms can be intrinsically linked, even if they are physically separated from one another. For example, if an electron in Arizona is measured to be “spin-up”, its corresponding entangled electron in New Zealand will instantly change its spin in a corresponding manner, as determined by the entanglement. Particles can even be entangled on opposite sides of the universe, seemingly undeterred by millions of light-years of spatial separation.
Entangled Quantum Dots
Entanglement can be fabricated by splitting a high-energy incident photon into a photon-photon pair that exists in an entangled state. Typical photon sources emit entangled photons at random times, but recent developments in quantum nanoscience have yielded quantum dots that can emit regular and predictable entangled pairs. This is once again owing to the large degree of tunability offered by quantum dots, as they can be manipulated to produce photons of exact wavelengths.
One of the fundamental problems posed by the quantum realm is the measurement problem, which is the very act of observing or measuring a system fundamentally alters its state. For example, electromagnetic radiation has been proven to exist as a wave or a particle, but if an observer tries to measure its state, the wavefunction collapses and only a discrete, quantized particle is recorded.
This problem echoes throughout the quantum realm, but entanglement offers a solution. By measuring one entangled particle, the observer can instantly know the state of the other entangled particle and the measurement problem is overcome. Quantum entanglement poses all sorts of uses in fields such as quantum cryptography, communications and computing. Quantum dots could pose the answer to synthesizing predictable and fine-tuned entangled pairs.
Nanoparticles for Medical Purposes
Nanoparticles such as quantum dots are being used for a range of medical applications. Their superior photophysical properties such as enhanced brightness, malleability and photostability make them a favorable alternative to traditional organic dyes. They have proven excellent tracers in medical imaging, but have been criticized for use within the human body due to their often-toxic inorganic components. Researchers have begun to synthesize non-toxic alternatives such as peptides, which occur naturally in organisms and therefore would likely produce safe dots for the body.
There are also concerns that the underlying quantum mechanics have not been tested enough and are not fully understood for use within the living human body. Quantum interactions between the dots and the body’s own cells may alter the nature of the dots, rendering them useless or even cause damage to the patient.
The Future of Quantum Nanoscience
Quantum nanoscience research is at the cross-section of quantum science and nanoscience: it enables scientists to develop nanotechnologies utilizing quantum mechanics to explore and use quantum effects in engineered nanostructures. This may aid the design of novel types of nanodevices and nanoscopic scale materials where functionality and structure of quantum nanodevices are described through superposition and entanglement.
Nanotechnology is ever-changing, and this evolutionary nanotechnology aims to improve existing processes, materials, and applications by scaling materials down to the nano-realm to fully exploit their unique quantum and surface phenomena.
In computing and electronics, microchips have already reached sub-100 nm, and the semiconductor industry is well on its way to becoming a nanotech-industry. By furthering our understanding of the quantum world, we will inherently develop more sophisticated and pertinent nanotechnologies.
References and Further Reading
Disclaimer: The views expressed here are those of the author expressed in their private capacity and do not necessarily represent the views of AZoM.com Limited T/A AZoNetwork the owner and operator of this website. This disclaimer forms part of the Terms and conditions of use of this website.